Fig latex inhibits the growth of pathogenic bacteria invading human ... - Nature.com
Abstract
Impaired wound healing is one of the most critical complications associated with diabetes mellitus. Infections and foot ulcers are major causes of morbidity for diabetic patients. The current treatment of diabetic foot ulcers, commonly used antibiotics, is associated with the development of bacterial resistance. Hence, novel and more effective natural therapeutic antibacterial agents are urgently needed and should be developed against the pathogenic bacteria inhabiting diabetic wounds. Therefore, the current study aimed to investigate the impact of fig latex on pathogenic bacteria and its ability to promote the healing process of diabetic wounds. The pathogenic bacteria were isolated from patients with diabetic foot ulcers admitted to Assiut University Hospital. Fig latex was collected from trees in the Assiut region, and its chemical composition was analyzed using GC‒MS. The antibacterial efficacy of fig latex was assessed on the isolated bacteria. An in vivo study to investigate the effect of fig latex on diabetic wound healing was performed using three mouse groups: nondiabetic control mice, diabetic mice and diabetic mice treated with fig latex. The influence of fig latex on the expression levels of β-defensin-1, PECAM-1, CCL2 and ZO-1 and collagen formation was investigated. The GC‒MS analysis demonstrated the presence of triterpenoids, comprising more than 90% of the total latex content. Furthermore, using a streptozotocin-induced diabetic mouse model, topical treatment of diabetic wound tissues with fig latex was shown to accelerate and improve wound closure by increasing the expression levels of β-defensin-1, collagen, and PECAM-1 compared to untreated diabetic wounds. Additionally, fig latex decreased the expression levels of ZO-1 and CCL2.
Introduction
A major health concern in the world today is diabetes mellitus (DM), which develops when the body is unable to produce or utilize insulin1. Globally, more than one in 10 adults are now living with diabetes. Moreover, there is a growing list of countries where one-in-five or even more of the adult population has diabetes2. According to the International diabetic federation (IDF) atlas the number of adults with diabetes is predicted to rise to 55 million by 20453. The different complications of the uncontrolled DM include retinopathy, cardiovascular diseases, neuropathy, nephropathy, and diabetic wound (DW)1,4. Patients with type 2 diabetes are more likely to suffer from these wounds, which are positively correlated with disease duration and the burden of complications5. Patients with uncontrolled diabetes have a high risk for diabetic foot ulcers (DFUs)6. Globally, it is estimated that 6.3% of diabetic patients have foot disease7, with North America generally having a higher regional incidence, which has been reported to reach 13% in some population studies8,9. It is anticipated that between 19 and 34% of people with diabetes will have a DFUs at some point in their lives6,10. Additionally, patients with DFUs are 2.5 times more likely to die than diabetic patients without foot disease; therefore, DFUs lead to significant mortality for affected patients11. DFUs affect 42.2% of diabetics and impose an economic burden on the health sector, that bears a cost of 3 billion dollars for the treatment every year12. Diabetic foot disease is one of the most challenging complications of diabetes to treat, and it has become a major cause of nontraumatic amputation13. In every 30 s, one person with diabetes is known to undergo lower extremity amputation somewhere in the world14. During diabetes, delayed wound healing is a result of different factors, including bacterial infections15. Indeed, 80% of patients with DFUs infected with pathogenic biofilm-forming bacteria need lower-limb amputation16. Hospitalization, amputation, and length of care have all been identified as major contributors to high health-care costs in the United States and elsewhere17,18. Treatments that promote speedy and complete healing of DFUs minimize the need for hospitalization, decrease the probability of amputation, and limit health-care costs19. DFUs complicated by diabetic foot infections (DFIs) are extremely probable20. The amputation rate in DFU patients is 38.4%21. Infection is a common (> 50%) side effect of DFUs22,23,24. New evidence emphasizes the significance of biofilm infection in the progression of nonhealing DFUs25. DFIs are polymicrobial in nature, and the growth mode of these microbes is complicated by the biofilm formation mechanism26,27. The inflammatory phase, which begins on the first day of wound injury and lasts for 10 days for wound repair, is a crucial stage in which colonizing pathogenic bacteria are encountered during tissue repair28. Most bacteria are genetically resistant to many antibacterial drugs. Because of the high morbidity rate, a large number of patients in hospitals are exposed to new infections due to the spread of pathogenic bacteria and drug resistance29,30. Therefore, multidrug-resistant bacteria contribute to delaying the steps of the healing process and result in the formation of chronic wounds28. Gangrene is one of the major and most severe consequences of bacterial infection associated with diabetes and is due to hyperglycemia leading to the failure of the immune response to control the spread of invading pathogens31,32. Polymicrobial biofilm aggregates have emerged as a major danger in the broader discipline of wound infection, as it is in this form that microbes acquire more resistance to host defenses and antibiotics, in addition to acquiring pathogenicity33,34. Pathogenic and commensal bacteria coaggregate synergistically in a pathogenic biofilm to establish a persistent infection35. Early identification and treatment of pathogenic microorganisms remain critical therapeutic goals that must be achieved36. Pathogenic bacteria such as Staphylococcus sp., Pseudomonas sp. and Bacillus species have been found to play a major role in attachment to catheters due to their ability to form biofilms37. Biofilms act as a mechanical barrier to antimicrobials and immune system cells and contribute to multidrug resistance38. Although Staphylococcus haemolyticus is considered a normal microbiota of the skin and an opportunistic pathogen associated with hospital-acquired infections, infections caused by it are increasing. Regardless of its low virulence profile, S. haemolyticus poses a serious risk to patients with DFUs due to its multidrug resistance39. Another concern with Staphylococcus infections is that some bacteria can produce biofilms to evade host immune systems and protect themselves against therapies, which play a crucial role in the virulence and pathogenicity of Staphylococcus spp. Nonhealing DFUs are distinguished by impaired wound healing associated with recurrent Staphylococcus aureus infection and unresolved inflammation40. A previous study tested S. haemolyticus strains isolated from bloodstream infections and discovered that 34% of them could form biofilms in vitro41. Moreover, multidrug-resistant Pseudomonas aeruginosa infection has become a challenge in clinical practice42,43,44. In the treatment of DFUs, commonly used antibiotics are linked to negative side effects, such as irritation, hyperpigmentation, histocompatibility, tissue rejection and the emergence of bacterial resistance45,46. Thus, empiric treatment for infected DFUs may fail if there is microbial resistance47. Moreover, the choice of the appropriate antibiotics for the infection is difficult; furthermore, coselection may involve resistance genes48. Globally, antibiotic resistance is a significant threat. According to the World Health Organization (WHO), "The world urgently needs to change the way it prescribes and uses antibiotics."49. Therefore, this problem can be solved by developing new medications with minimal or no side effects and without developing new resistant microbes. Consequently, the usage of natural compounds in pharmaceuticals has greatly expanded. According to one of the World Health Organization (WHO) performed investigations, approximately 80% of the global population received medications containing chemicals produced from natural compounds50,51,52,53 for a wide range of infections. Many phytochemicals are antibacterial and play an integral role in healing therapies54. The biomolecular compounds generated and extracted from plants are a valuable source of antibacterial and anti-biofilm-forming pathogenic bacteria55. Plants have been used for therapeutic purposes because they are associated with fewer toxic effects and are more economical than manufactured therapies56,57.
The fluid in some laticiferous plants called latex contains bioactive compounds with valuable antibacterial and antioxidant effects. Latex is a biological fluid secreted by a wide range of plants and is composed of several types of metabolites, such as polyisoprene and sugars. The protein compounds of latex (peptidases, lipases, chitinases, steroids and numerous secondary metabolites) are commonly found in various latex fluids. In addition to the industrial importance of plant latex as a rubber source, as from the para rubber tree (Hevea brasiliensis), latex is a component of plant defense against microbes58. The stored latex in specialized cells called laticifers is secreted in response to any plant physical damage or wounding58.
In contrast to most latex-bearing plants, which store small, limited amounts of latex, the fruit of the fig tree (Ficus carica) exudes a high amount of latex. From ancient times to the present, fig latex has been used in India for people who suffer cracks in the mouth or on the lips or tongue because it is an excellent tonic. This effect may be due to fig latex enzymes such as ficin, proteases, lipodiastase, and amylase59. Plant latex is frequently applied topically to a variety of wounds and plays a key role in wound care60,61,62. Many endogenous proteases are involved in the various stages of wound healing during the physiological healing process; similarly, latex proteases used to treat wounds will act on various stages of wound healing63. For instance, procoagulant and thrombin-like proteases function to restore hemostasis during the early stages of wound healing. In the later stages of wound healing for debridement, plasmin-like and other ECM-degrading proteases assist, and some mitogenic proteases aid in cell proliferation and angiogenesis. Plant latex collagenolytic and other ECM-degrading proteases could play a role in the remodeling of collagen and other extracellular matrix (ECM) components62.
In addition to the increase in health complications while using chemically synthetic drugs, the large-scale use of antibiotics leads to different strains of multidrug-resistant bacteria. The latex of figs has been applied on a large scale in the treatment of warts, skin ulcers and sores and can be taken as a purgative and vermifuge59. Fig latex is useful for milk coagulation and can be used as a lipid-lowering drug due to the presence of triterpenoids64. Fig latex is considered a restorative natural material that helps in quick recovery after prolonged illness65. Due to its antimicrobial activities, low toxicity, and availability, fig latex represents a remedy for several health complications66. Nevertheless, no published work has demonstrated the antibacterial activities of fig latex against the pathogenic bacteria inhabiting diabetic wounds. Thus, the current study is a pioneer study evaluating the potential impact of fig latex on pathogenic bacteria isolated from human DFUs in vitro. Furthermore, the study aimed to clarify the efficacy of fig latex in accelerating the healing process of diabetic wound skin tissues in a streptozotocin (STZ)-induced diabetic mouse model in vivo by monitoring the expression of some proteins related to the healing process, such as β-defensin (antibacterial protein) and zonula occluden-1 (ZO-1), using ELISA. Additionally, the expression levels of CCL2 and PECAM-1 were detected using immunohistochemistry (IHC).
Results
Gas chromatography‑mass spectrometry (GC–MS) analysis of fig latex
The GC‒MS analysis of fresh fig crude latex showed the presence of twenty-four bioactive compounds with concentrations more than 0.1% of the total latex contents. The most abundant compound with the highest percentage was taraxasterol acetate (more than 50% of the total latex content). Moreover, the olean-12-en-3-ol, acetate, (3.beta.)- was detected and represented 32% of the total latex content. Finally, 9,19-cyclolanost-24-en-3-ol, (3.beta.)- (3.999%) and Lanosta-8,24-dien-3-ol, acetate, (3.beta.)- (3.346%) were detected. All four major chemical constituents represented 90% of the latex. Other active compounds, along with their concentration (peak area %) and retention time (RT), are presented with the chromatogram in Fig. 1, and the chemical components obtained from the GC‒MS analysis are presented in detail in Table 1.
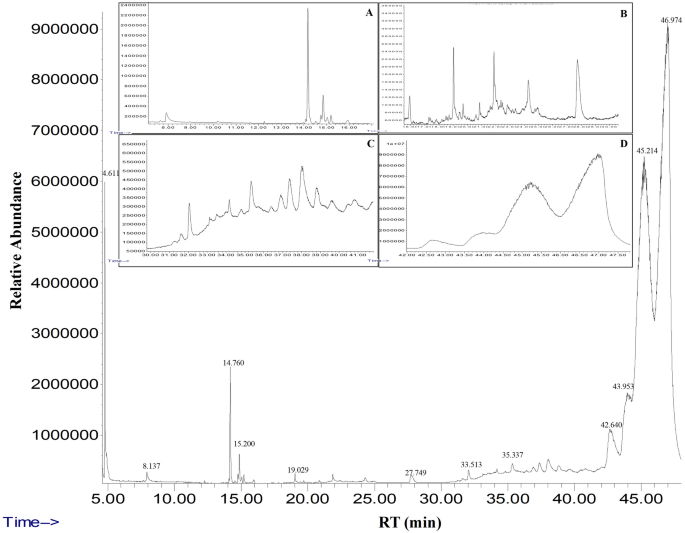
Gas chromatography and mass spectrometry chromatogram of fig latex analysis. The analysis demonstrated several peaks with different retention times to 47 min of twenty-four active constituents. A, B, C and D are zoomed regions of the chromatogram, RT, retention time.
Fig latex exerts antibacterial activity using the well diffusion method
After the bacteria were obtained from DFUs using a swab method, the bacterial community was screened. We isolated many pure strains using the streak plate method, spreading bacteria in different culture media, such as MSA for gram-positive bacteria and MacConkey agar for gram-negative bacteria. The antibacterial activity of fresh fig latex was tested against five DFU biofilm-forming bacterial isolates, Staphylococcus haemolyticus AUMC B-331, two Gram-negative Pseudomonas sp., Bacillus sp., and Paenibacillus sp., to determine the efficacy of the latex as an antibacterial drug. The diameter of the inhibition zone is shown in Fig. 2. The largest clear zones formed by fresh fig latex were 32, 30, and 28 mm, against St. haemolyticus AUMC B-331, Bacillus sp., and Paenibacillus sp., respectively, whereas the smallest clear zone observed was 18 mm against Pseudomonas sp. MS2, and there was no significant effect with no clear zone or growth inhibition against Pseudomonas sp. MS4. Stored latex showed less activity than fresh crude latex, as presented in Table 2.
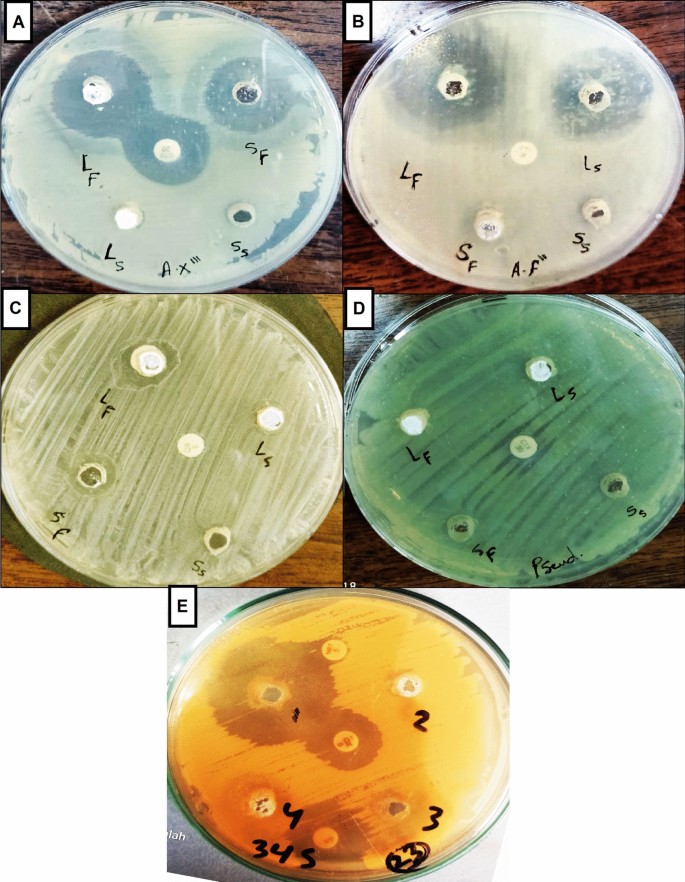
Inhibition zone showing the antibacterial activity of fresh fig latex on bacteria isolated from diabetic wounds. Causing a clear zone with diameter against the pathogenic bacteria (A) Paenibacillus sp., (B) Bacillus sp., (C) Pseudomonas sp. MS2, (D) Pseudomonas sp. MS4 and (E) Staphylococcus haemolyticus AUMC b-331.
Determination of the minimum inhibitory concentration (MIC) and minimum bactericidal concentration (MBC)
The MIC value was found to be 250 mg/mL, demonstrating a promising effect of fresh fig latex as a natural antibacterial agent. According to broth macrodilution results, the MIC was 250 mg/ml for St. haemolyticus AUMC B-331, Bacillus sp., Pseudomonas sp. MS2, and Paenibacillus sp., and the MBC was 500 mg/mL; the MIC of fig latex for Pseudomonas sp. MS4 was not reported (Fig. 3).
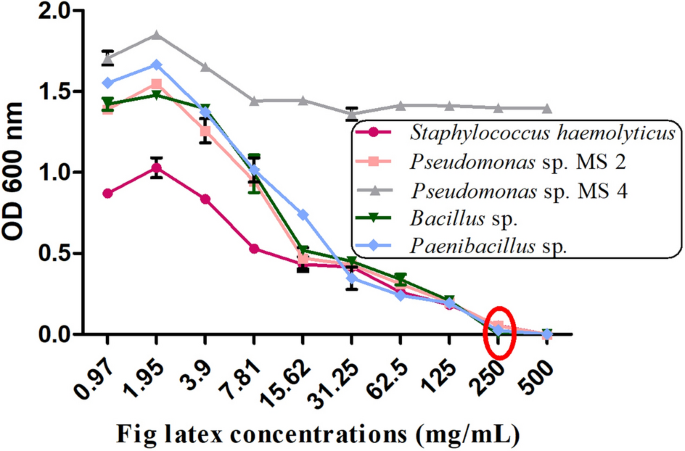
Minimum inhibition concentration (MIC) of fig latex for gram-positive and gram-negative bacteria. MIC reduction is presented as the decrease in the OD600 of the bacterial suspension treated with latex at various concentrations (0.97, 1.95, 3.9, 7.81, 15.62, 31.25, 62.5, 125, 250 and 500 μg/ml) against St. haemolyticus, Ps. MS2, Ps. MS4, Bacillus sp. and Paenibacillus sp. showing the MIC at 250 mg/ml with a red circle.
Fig latex reduces bacterial biofilm formation
The results of the plate shown in Fig. 4A were quantified using a microplate reader at OD 600 nm, and it was revealed that there were five isolates forming the biofilm: Staphylococcus haemolyticus AUMC B-331, Pseudomonas sp. MS2, Pseudomonas sp. MS4, Bacillus sp., and Paenibacillus sp. Low, moderate, and high bacterial biofilm-forming ability was interpreted when OD600 nm was < 1, 1–2.9 and > 2.9, respectively. Accordingly, Pseudomonas sp. MS4 showed high biofilm-forming ability, while Pseudomonas sp. MS2 and St. haemolyticus showed moderate ability. On the other hand, Bacillus sp. and Paenibacillus sp. had low biofilm-forming ability. In Fig. 4B, the ability of fig latex to reduce the bacterial biofilm formed by these isolates is illustrated. Notably, it was found that fresh fig latex at the MIC had the ability to significantly reduce the biofilm formed by four isolates out of the five tested, Staphylococcus haemolyticus AUMC B-331, Pseudomonas sp. MS2, Bacillus sp., and Paenibacillus sp. However, it had no significant activity against Pseudomonas MS4 sp. The percent biofilm inhibition after treatment with fig latex is shown in Fig. 4C. Additionally, after treatment with fig latex, the biofilm formed by Staphylococcus haemolyticus AUMC B-331 was decreased by 73%, that formed by Pseudomonas sp. MS2 was decreased by 37%, that formed by Paenibacillus sp. was decreased by 39% and that formed by Bacillus sp. was decreased by 67%. Additionally, fig latex inhibited the biofilm formed by Pseudomonas sp. MS4 by only 11%, showing that there was no significant effect of latex on Pseudomonas sp. MS4.
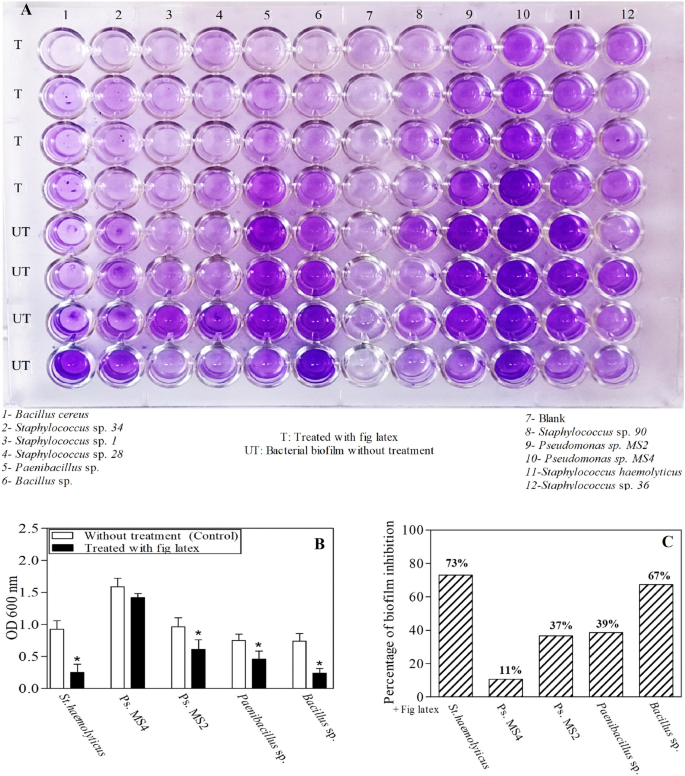
The impact of fig latex on bacterial biofilm using a microtiter plate biofilm assay against five pathogenic bacterial isolates from diabetic foot ulcers. (A) Microtiter plate showing the differences in the color intensity; (B) Quantitative determination of the optical density of the biofilm from the isolated bacteria as control (open bars) and after treatment with latex (closed bars); (C) Percentage of the antibiofilm activity of fig latex against the bacterial isolates (St. haemolyticus, Ps. MS2, Ps. MS4, Bacillus sp. and Paenibacillus sp.).
Survival curve of DFU pathogenic bacteria in the presence of fig latex
The survival curve test of isolated bacteria demonstrated a slightly similar increase in growth over 24 h. The growth curves were decreased in the case of the treatment of the bacterial culture with the MIC of fresh fig latex after nearly 8 h, with a significant growth inhibition starting at 16 h of culture with fig latex, as demonstrated in Fig. 5A–C, E. In contrast, the growth curve of Pseudomonas sp. MS4 did not show any significant decrease after the treatment of the culture with fig latex, suggesting that it is a strain resistant to fig latex (Fig. 5D).
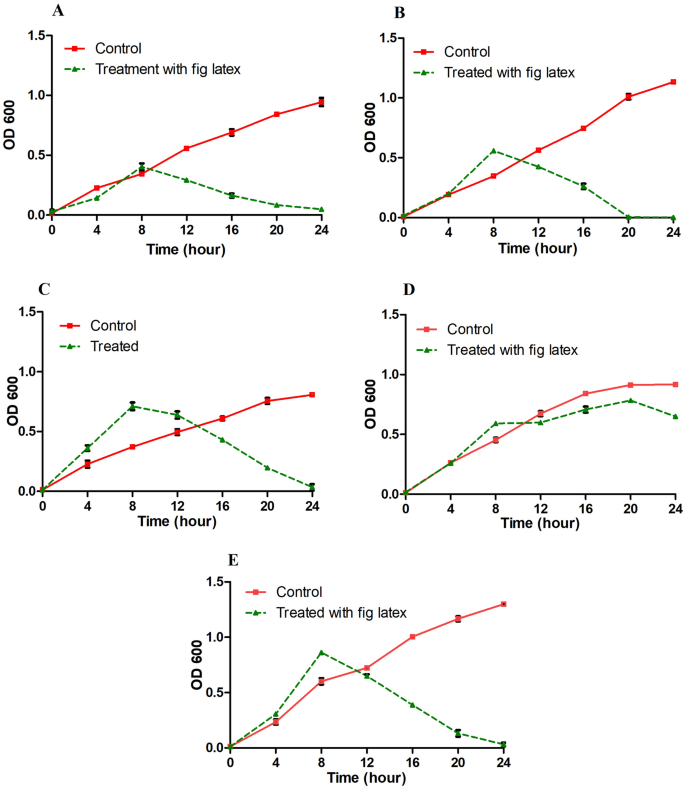
Survival curve of DFU pathogenic bacteria in the presence of fresh fig crude latex. The curves represent the bacterial isolates supplemented with the MIC of fresh fig latex in TSB media (dashed line) and show a significant decrease in OD600 for (A) Paenibacillus sp., (B) Bacillus sp., (C) Pseudomonas sp. MS2, and (E) Staphylococcus haemolyticus AUMC B-331 but not for (D) Pseudomonas sp. MS4. The curves represent the bacterial isolates in TSB media without latex (solid line) and show an increase in OD at 600 nm. The results are the mean from triplicate independent measurements.
Fig latex enhances the healing process of diabetic wounds
The in vivo experiment on the mouse model, shown in Fig. 6A, monitored the morphological changes occurring in the three animal groups at each time point from Day 0 to Day 15 post-wounding. It was noted that in the diabetic animals, the healing process of wounds was delayed, and abscesses were found in the diabetic wounds with no healing compared to the healing process of wounds in the control group. Most importantly, topical application of fig latex on the diabetic wounds twice daily improved and accelerated the wound closure near that found in the control group. Figure 6B shows the wound size starting from 8 mm in the three animal groups at Day 0 post-wounding. The diabetic group showed a significant increase in the wound diameter (4 mm) (impaired healing) compared to the wound diameter (0 mm) in the control group at Day 15 post-wounding. Interestingly, when the wounds were topically treated with fresh fig latex, the wound diameters (0 mm) were similar to those found in the control group. The percentage of wound closure was calculated from the accumulated data (Fig. 6C). The diabetic animals exhibited a significant decrease in the percentage of wound closure compared to the control nondiabetic group. Amazingly, when the diabetic wounds were topically treated with fresh fig latex, the percentage of wound closure was significantly enhanced a level similar to that found in the control group.
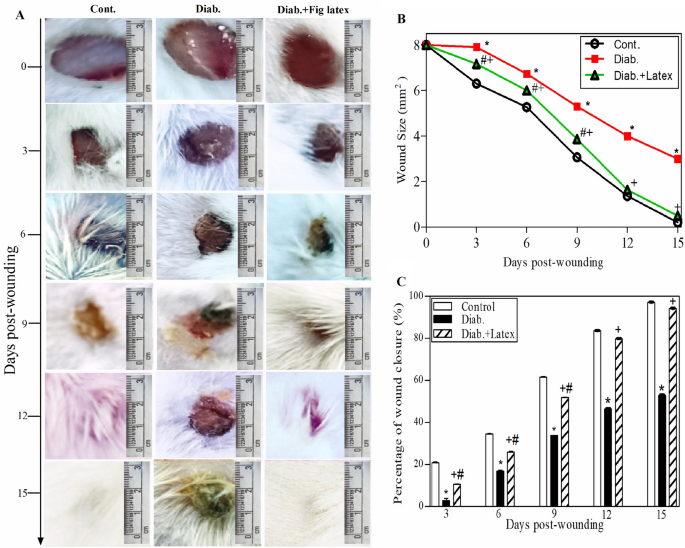
Effect of fig latex on wound healing and wound closure in diabetic mice. (A) Photographs showing the effect of fig latex on accelerating the healing process in diabetic wounds at each time point from Day 0 (the day of wound creation) until Day 15 (final point). (B) The accumulated data from three individual mice in each group at each time point post-wounding represent the change in diameter (mm). (C) The accumulated results from three animals in each group for each time point post-wounding were calculated by the previously mentioned equation in the Methods section, and the results show the percentage of wound closure. The data are expressed as the mean ± SEM. *P < 0.05, Diab. versus cont., +P < 0.05, diabetic mice treated with Latex versus cont., #P < 0.05, diabetic treated with Latex versus diabetic group.
Fig latex restores collagen formation in the wounded skin tissues of diabetic mice
Figure 7A–E shows representative pictures of wounded skin tissues stained with Sirius red to monitor collagen deposition. In Row A, at Day 3, the three animal groups showed similar collagen deposition. However, from Row B to E, it was found that from Day 6, the expression of collagen began to obviously increase in the control group over time until reaching the maximal expression at Day 15 post-wounding. However, the diabetic animals showed an obvious decrease in the expression of collagen compared to the control animals. Most importantly, the treatment of diabetic mice with fig latex restored collagen expression to levels similar to those found in the control group. Using ImageJ software, the collagen expression in three animals per group was quantified, and accumulated data are presented in Fig. 7F. From Day 6 to Day 15 post-wounding, both control and fig latex-treated diabetic animals demonstrated similar expression of collagen in wounded skin tissues. Nevertheless, the diabetic animals exhibited a significant decrease in the expression of collagen.
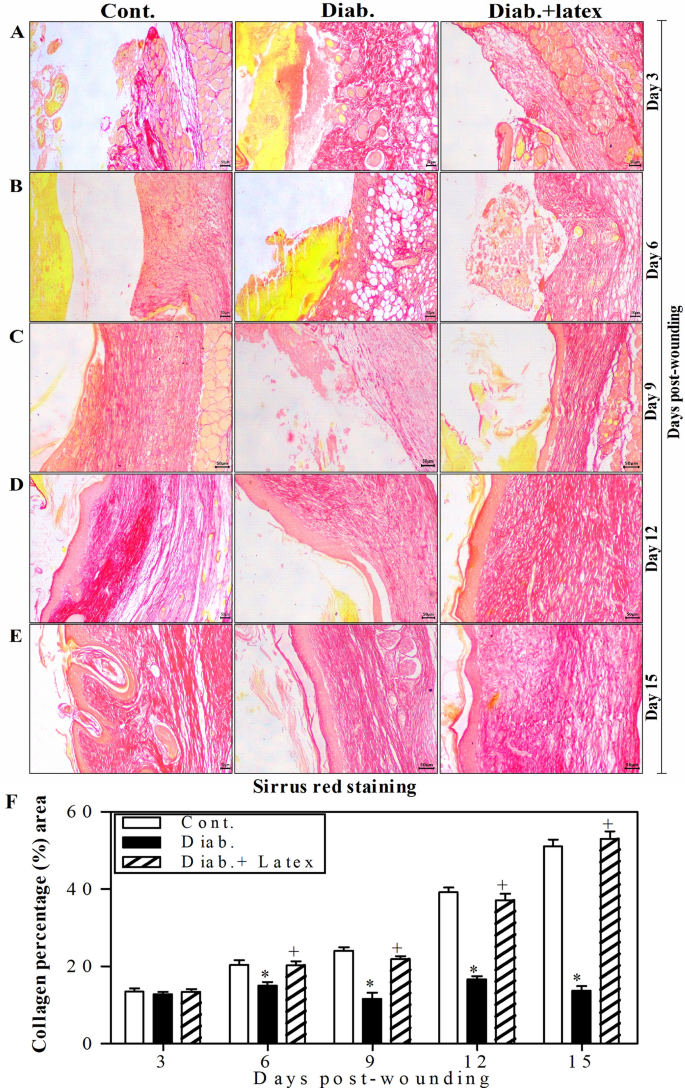
Collagen deposition in the wounded skin tissues stained with Sirius red. Collagen formation (red color) was detected in the wounded skin tissues of the three animal groups using Sirius red staining. One representative experiment is shown at 3 (A), 6 (B), 9 (C), 12 (D) and 15 days (E) post-wounding. (F) The percentages of collagen formation were quantified in the three animal groups, and the accumulated data from three animals from each group are expressed as the mean percentage of collagen formation ± SEM in control mice (open bars), diabetic mice (closed black bars) and diabetic mice treated with fig latex (hatched bars). *P < 0.05, diabetic versus control; and +P < 0.05, diabetic-treated with fig latex versus diabetic animals.
Fig latex enhances vascularization in wounded diabetic skin tissues
Figure 8A–E displays representative pictures of the immunohistochemical (IHC) expression of platelet endothelial cell adhesion molecule (PECAM-1) as a marker of vascularization in wounded skin tissues. The diabetic animal group showed a decrease in the expression of PECAM-1 from Day 3 to 15 post-wounding, with a mild positive reaction compared to those found in the control animals, which exhibited a strong positive reaction of PECAM-1 expression. Amazingly, the wounded tissues of diabetic skin mice treated with fig latex exhibited a moderate reaction compared to the control group. Using ImageJ software, accumulated data for PECAM-1 expression were quantified and are presented in Fig. 8F. The diabetic mice showed a significant decrease in the expression levels of PECAM-1 from Day 3 to Day 15 post-wounding. In contrast to the diabetic group, both control and fig latex-treated mice exhibited a significant increase in the expression of PECAM-1 from Day 3 to Day 15 post-wounding.
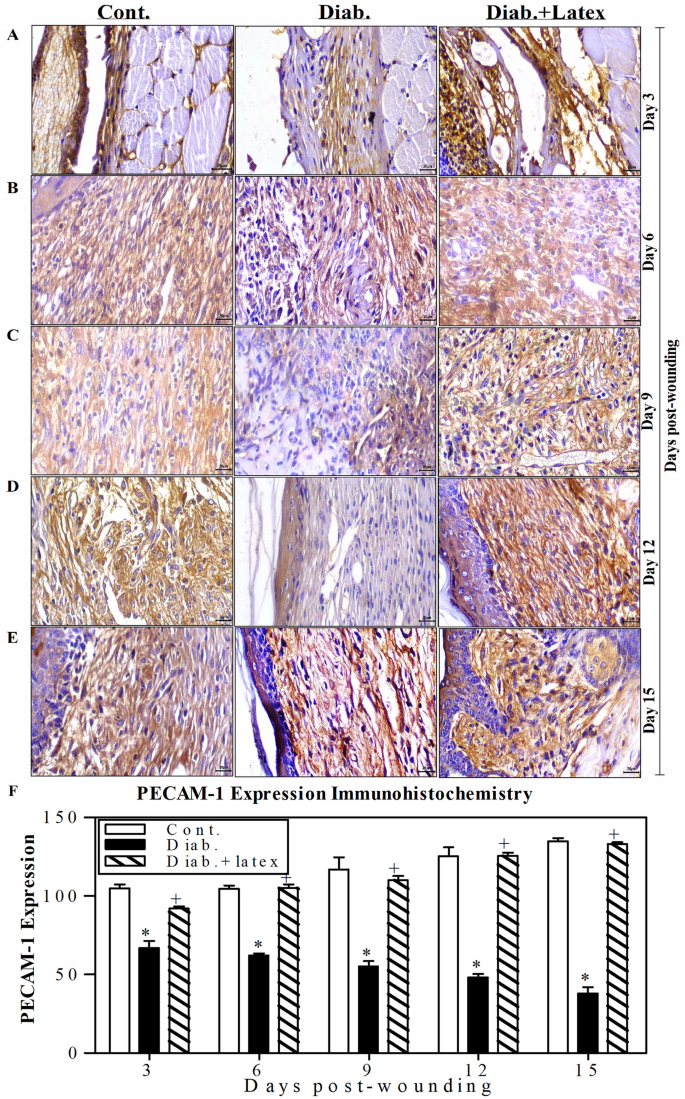
Effects of fig latex on the expression levels of PECAM-1 in wounded diabetic skin tissues. The expression levels of PECAM-1 were detected in the wounded skin tissues of the three animal groups using anti-PECAM-1 and IHC assays. One representative experiment is shown at 3 (A), 6 (B), 9 (C), 12 (D) and 15 days (E) post-wounding. (F) The PECAM-1+ cells were quantified in the three animal groups, and the accumulated results from three animals from each group are expressed as the mean percentage of PECAM-1+ cells ± SEM in control mice (open bars), diabetic mice (closed black bars) and diabetic mice treated with fig latex (hatched bars). *P < 0.05, diabetic versus control; and +P < 0.05, diabetic-treated with fig latex versus diabetic animals.
Fig latex decreases the expression level of inflammatory CCL2 in wounded diabetic skin tissues
Figure 9A–E shows one representative image of the expression of CCL2 (an inflammatory chemokine). Predominantly, it was noticed that there was increased CCL2 expression in diabetic wounds from Day 3 to 15 after wounding compared to the CCL2 expression in the wounds of the control group, suggesting the presence of the severe complications and protein expression dysregulation associated with diabetes. Topical treatment of diabetic mice with fig latex decreased CCL2 expression to levels similar to those found in the control group. The accumulated data from three animals per group (Fig. 9F) were quantified using ImageJ software. The data demonstrated a gradual significant elevation in CCL2 expression from Days 3 to 15 post-wounding. Nevertheless, the expression levels of CCL2 were significantly decreased in the wounded tissues of the control and fig latex-treated groups with a notable mild reaction.
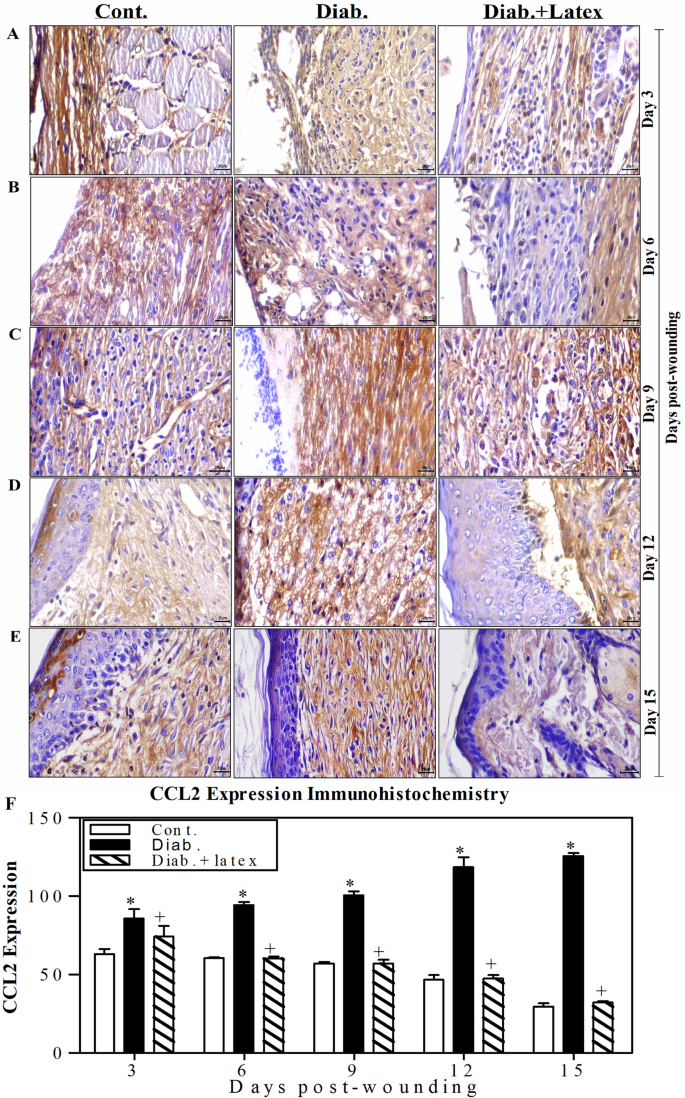
Topical application of fig latex restored the expression levels of CCL2 in wounded diabetic skin tissues. (A, B, C, D and E) show one representative experiment of CCL2 expression in the control mice, diabetic mice and diabetic mice treated with latex at Days 3, 6, 9, 12 and 15 post-wounding. (F) The CCL2+ cells were quantified in the three animal groups, and the accumulated results from three animals from each group are expressed as the mean percentage of CCL2+ cells ± SEM in control mice (open bars), diabetic mice (closed black bars) and diabetic mice treated with fig latex (hatched bars). *P < 0.05, diabetic versus control; and +P < 0.05, diabetic-treated with fig latex versus diabetic animals.
Fig latex accelerates the healing process of diabetic wounds via the restoration of the expression levels of β-defensin-1 and ZO-1
It was concluded that fig latex inhibits pathogenic growth in vitro; hence, the expression levels of an antibacterial protein (β-defensin) and a tight junction protein (ZO-1) were monitored using ELISA and are shown in Fig. 10A, B, respectively. Our results showed decreased levels of β-defensin-1 and elevated levels of ZO-1 in diabetic mice compared to control animals. Interestingly, fig latex-treated diabetic mice exhibited significantly restored levels of β-defensin-1 and ZO-1 compared to vehicle-treated diabetic animals.
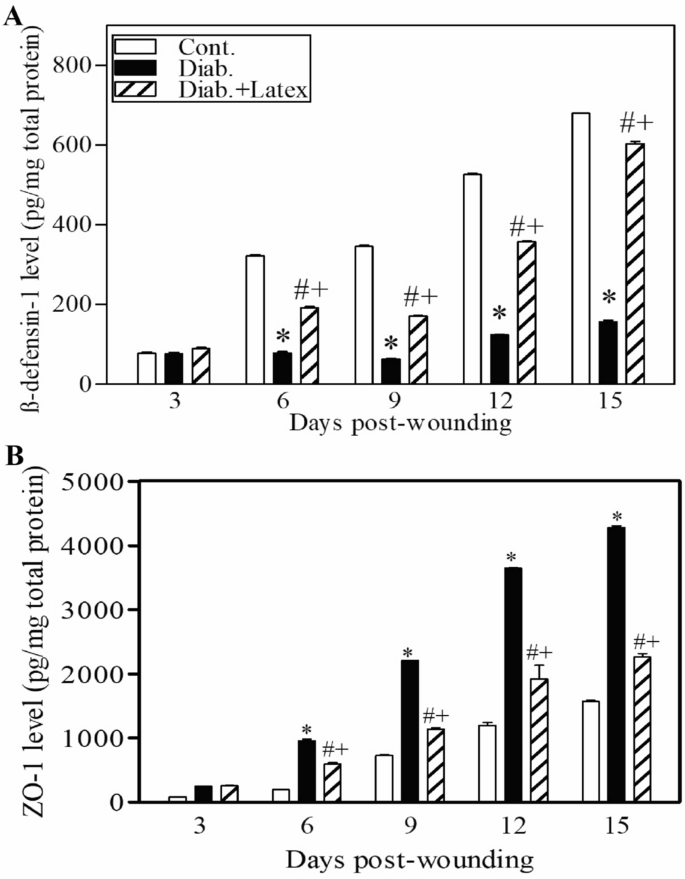
Topical application of fig latex altered the expression of β-defensin-1 and ZO-1. The levels of β-defensin-1 (A) and ZO-1 (B) in the wounded skin tissues of control mice (open bars), untreated diabetic mice (closed black bars), and diabetic mice treated with fig latex (hatched bars) were measured using ELISA. The collected data from three mice in each group are expressed as the mean ± SEM (n = 3). *P < 0.05 for diab. versus cont.; +P < 0.05 for diab. treated with fig latex versus cont.; and #P < 0.05 diab. treated with fig latex versus diab. (ANOVA with Tukey's post-test).
Discussion
Several lines of evidence were used to demonstrate that fig latex exhibited major compounds with powerful biological activities, especially for inhibiting the biofilm formed by the pathogenic bacteria inhabiting diabetic wounds, and their pivotal role for accelerating the healing process. The GC‒MS analysis of fig latex revealed that crude fresh latex contains several phytochemical components, such as terpenes, coumarins, flavonoids and alkaloids67. Taraxasterol acetate, which is known as pentacyclic triterpenoid68, was only identified in 2006 by Teixeira et al. screening in fig leaves in Portugal67. This important compound was detected for the first time and made up more than 50% of the total latex content in fig latex collected in Egypt and has never been recorded before in any previous analysis of fig latex; this may be due to the difference in the geographic distribution. Another important compound is the triterpenoid olean-12-en-3-ol, acetate, also named β-amyrin acetate69, which was quantified in the fig latex analysis and represented 32% of the total latex content. Interestingly, some fig latex components identified in GC‒MS analyses have been found to have antimicrobial activities, such as α-gurjunene70, nonadecane71, germacrenes, pentadecane72,73, cis-13-eicosenoic acid, tetracosahexaene74, 2,5-di-tert-butyl-p-quinone75, cis-vaccenic acid76, and lanosta-8,24-dien-3ol, acetate (3.beta. also displayed antifungal and antibacterial activity77. Other components, such as humulene78, elemene79, 1-[5-(2-methylphenoxy)pentyl]piperidine, α-amyrin acetate80, and β-amyrin acetate81, have shown anti-inflammatory activities, and high-concentration taraxasterol acetate has been shown to have significant antibacterial, anti-inflammatory and anticancer activities82. Some of these compounds were identified in our GC‒MS analysis and were detected in several previous GC‒MS analyses of fig latex as previously described83,84; these compounds are considered antibacterial phytochemicals. Hence, in the current study, the antibacterial effect of fig latex was due to the fact that more than 83% of the total latex content was taraxasterol acetate and β-amyrin acetate. Most importantly, our data revealed the antibacterial activity of fresh fig latex against pathogenic bacteria. Similarly, Aref et al.84 proposed that this may be caused by the fact that these two major compounds exhibited similar antibacterial activity against both gram-positive bacteria, especially Staphylococcus sp., and gram-negative bacteria85,86,87. Moreover, fig latex was shown to be a therapeutic agent for the complications associated with diabetes, such as an impaired healing process due to the inhibition of insulin sensitivity leading to hyperglycemia88, as indicated by the results of the decrease in glucose levels in the mouse model we used after topical treatment with fig latex. In the results of the in vitro study of the antibacterial activity, fresh fig latex showed significant activity against the tested biofilm-forming pathogenic bacteria isolated from diabetic wounds, with MICs of 250–500 mg/ml. This result was also observed in several previous studies, one of them against oral bacteria89,90, with MICs ranging from 500 to 750 mg/ml. In addition, the study performed by Aref et al.91 investigated the antibacterial effect of fig latex on Escherichia coli, Proteus mirabilis, and Staphylococcus aureus with an MIC of 5 mg/ml. Similar to the results in the study performed in 2014 by Rashid et al., the antibacterial activity of fig latex against Staphylococcus aureus, Streptococcus pyogenes, Escherichia coli, Salmonella typhi, Pseudomonas aeruginosa, and Klebsiella pneumoniae was better than that of gentamycin92. Additionally, fresh latex was more effective than stored latex, which confirms the results of previous studies93. Our results demonstrated a lack of antibacterial activity of fig latex against the bacterial strain Pseudomonas sp. Ms4 (Resistant). The biofilm assay showed that fig latex decreased the biofilm formed by the tested strains isolated from diabetic wounds, especially Staphylococcus sp., and it was shown in preclinical trials that fig latex was highly successful for clearing biofilm-forming Staphylococcus species94. Antibiotics lose their ability to fight bacteria that are protected inside biofilms. In wound treatment, one of the remedies is to boost the antibiotic concentration by a thousand-fold. Another option is to target the biofilm directly, allowing the antib...
Comments
Post a Comment