Ginsenoside Rg3 enriches SCFA-producing commensal bacteria to ... - Nature.com
Abstract
The microbiota-associated factors that influence host susceptibility and immunity to enteric viral infections remain poorly defined. We identified that the herbal monomer ginsenoside Rg3 (Rg3) can shape the gut microbiota composition, enriching robust short-chain fatty acid (SCFA)-producing Blautia spp. Colonization by representative Blautia coccoides and Blautia obeum could protect germ-free or vancomycin (Van)-treated mice from enteric virus infection, inducing type I interferon (IFN-I) responses in macrophages via the MAVS-IRF3-IFNAR signaling pathway. Application of exogenous SCFAs (acetate/propionate) reproduced the protective effect of Rg3 and Blautia spp. in Van-treated mice, enhancing intracellular Ca2+- and MAVS-dependent mtDNA release and activating the cGAS-STING-IFN-I axis by stimulating GPR43 signaling in macrophages. Our findings demonstrate that macrophage sensing of metabolites from specific commensal bacteria can prime the IFN-I signaling that is required for antiviral functions.
Introduction
Cumulative evidence supports that the intestinal microbiome can inhibit viral infections both locally and systemically through the release of microbial metabolites [1,2,3,4]. For example, Winkler et al. demonstrated that Clostridium scindens can modulate prompt type I interferon (IFN-I) responses to prevent Chikungunya virus from infecting and disseminating in host blood monocytes via primary to secondary bile acid transformation [4]. In addition, one Clostridium orbiscindens-derived flavonoid degradation product, desaminotyrosine, has been shown to augment IFN-I responses in pulmonary macrophages and protect mice from influenza A virus (IAV) infection [2].
Short-chain fatty acids (SCFAs) including acetate, propionate and butyrate are mainly generated in the colon by bacterial fermentation of undigested complex carbohydrates [5]. SCFAs are generally considered to elicit anti-inflammatory properties either by binding to the G protein-coupled receptors GPR41 (also known as FFAR3) and GPR43 (also known as FFAR2), or by inhibiting histone deacetylases in different cell types [6, 7]. SCFAs can impact viral infections both in vitro [8] and in vivo [9]. For instance, acetate or dietary fiber-derived butyrate can exert protective effects against IAV infection by inducing IFN-I responses [10] or reducing neutrophil infiltration into the airways [11].
Although the gut microbiome has been described to play a role in regulation of innate and adaptive antiviral immune responses [12,13,14], very little is known about the interplay between SCFA-producing commensal bacteria, the host immune system and infecting enteric viruses. In a previous study we demonstrated that the intestinal microbiome primes IFN-I induction in mononuclear phagocytes to antagonize systemic encephalomyocarditis virus (EMCV) infection via an IFNAR- and STAT1-mediated signaling pathway [15]. Although the acetogenic bacterium Blautia coccoides has been identified to confer protection against enteric viruses in an IFN-I-dependent manner, whether SCFAs can regulate IFN-I signaling in mononuclear phagocytes remains unknown. Furthermore, a mechanistic understanding of the signaling pathways linking host antiviral IFN-I immune responses and microbial-derived SCFAs during enteric virus infection is still lacking.
Ginsenosides are the main active components of ginseng, an ancient herb that has been used extensively worldwide as a natural tonic [16]. Ginsenosides Rb1 and Rg1 have previously been shown to restrict murine norovirus (MNV) replication in vitro [17]. Here, we describe notable enrichment of SCFA-producing commensal bacteria Blautia spp. in mice following ginsenoside Rg3 (Rg3) consumption. Acetate and propionate, which are known metabolites of Blautia spp. primed IFN-I-mediated innate antiviral immunity to enteric viral challenge at mucosal and extraintestinal sites via GPR43-cGAS-STING signaling.
Results
Rg3 elicits protection against local and systemic enteric virus infection by regulating the intestinal microbiome
To assess the antiviral effects of Rg3 in vivo, groups of C57BL/6J mice (referred to as WT B6 hereafter) were orally administered Rg3 for 3 consecutive days, inoculated with MNV-1 by the peroral route, and viral burden in intestinal tissues was determined at 1 day post-infection (dpi). Rg3 treatment led to diminished viral replication in the duodenum, jejunum, ileum, colon, mesenteric lymph nodes (MLNs) and also resulted in decreased fecal viral shedding relative to the untreated controls (Fig. 1a). Additionally, Rg3 treatment of mice for 7 or 14 days prior to infection resulted in significantly lower viral titers in the intestinal tissues, MLNs and feces compared with untreated controls, in a time-course dependent manner (Supplementary Fig. S1A). This phenotype was not due to a direct virostatic effect of Rg3, since Rg3-pretreated primary bone marrow-derived macrophages (BMDMs) showed no reduction of viral titers in vitro after MNV-1 infection at different multiplicities of infection (MOI) (Supplementary Fig. S1B).
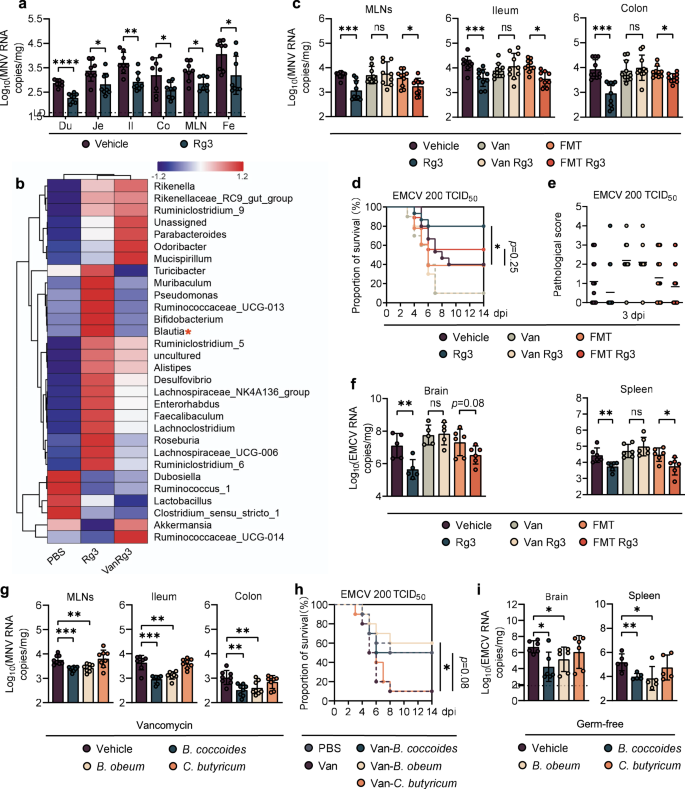
a Viral burden analysis at 1 day post-infection (dpi) of duodenum (Du), jejunum (Je), ileum (Il), colon (Co), mesenteric lymph nodes (MLNs) and feces (Fe) collected from vehicle-treated or Rg3-treated mice infected with 2 × 107 TCID50 MNV (n = 8). b Heatmap of the relative abundance of bacteria in feces from MNV-infected mice at 0 dpi (n > 3). c Viral burden analysis of MLNs, ileum and colon tissues collected at 1 dpi from vehicle-, Rg3-, Van-, Van-Rg3-, FMT- treated or FMT-Rg3-treated mice that were orally inoculated with 2 × 107 TCID50 MNV (n = 8). Survival curve (d) and pathology scores (e) from groups of differently treated mice i.p. infected with 2 × 102 TCID50 of EMCV (n = 15–18). f Viral titers in brain (left) and spleen (right) collected from differently treated mice at 3 dpi following i.p. infection with 1 × 103 TCID50 of EMCV (n = 6). g Viral burden at 1 dpi in MLNs, ileum and colon tissues collected from Van-treated mice that were subsequently colonized with B. coccoides, B. obeum, C. butyricum and then inoculated orally with 2 × 107 TCID50 MNV (n = 8). h Survival curves from groups of PBS-, Van-treated and bacteria-colonized WT mice that were i.p. infected with 2 × 102 TCID50 of EMCV (n = 10). i Viral burden at 3 dpi in brain and spleen of germ-free (GF) mice colonized with B. coccoides, B. obeum or C. butyricum for 48 h and then i.p. infected with 2 × 102 TCID50 EMCV (n = 5–6). Broken lines indicate the limit of detection (LD). Plotted data represent the mean ± s.d. Unpaired two-tailed Student's t tests were used for comparison of means between treatment groups. *p <0.05; **p < 0.01; ***p < 0.001; ****p < 0.0001; ns not significant.
To test if the protective antiviral effects exerted by Rg3 in vivo were due to alterations of the intestinal microbiome, we first performed 16S rRNA sequencing on stools from PBS- or Rg3-treated mice. Global community composition at the family level indicated that Rg3 administration induced a considerable increase in the proportion of Lachnospiraceae and Bifidobacteriaceae together with a significant reduction in the numbers of operational taxonomic units (OTUs) belonging to the Akkermansiaceae and Lactobacillaceae families (Supplementary Fig. S1C). In particular, well-known SCFA-producers including Blautia, Faecalibaculum and Lachnoclostridium were the main enriched genera in the Rg3-treated mice compared with their PBS-treated counterparts (Fig. 1b).
We performed microbiota depletion studies to further confirm the hypothesis that Rg3 alterations to the intestinal flora confers protection against enteric virus infection. Groups of WT B6 mice were gavaged with vancomycin (Van), neomycin (Neo), ampicillin (Amp), or metronidazole (Metro) for 5 days and subsequently treated with Rg3 for 3 days before MNV-1 inoculation. Depletion of the gut microbiota by antibiotic treatment was observed as a significant reduction in 16S rRNA gene copies (Supplementary Fig. S1D), and a greatly altered enteric bacterial community composition before infection (Supplementary Fig. S1E). Van was selected for subsequent studies due to its high efficiency in depleting Gram-positive, anaerobic, fermentative, SCFA-producing spp. of the Lachnospiraceae family (Fig. 1b).
The MNV-1 inhibitory effect of Rg3 treatment was abolished when mice were pretreated with Van, whereas reconstitution of the gut flora by fecal microbiota transplantation (FMT) from antibiotic-naive, uninfected mice resulted in decreased MNV-1 titers in the ileum, colon and MLNs of the Rg3-treated mice (Fig. 1c). As expected, FMT restored the 16S rRNA gene copy numbers that had been substantially reduced by Van treatment (Supplementary Fig. S1F).
We tested the protective effect of Rg3 treatment against EMCV challenge, a more severe viral infection model that results in severe encephalitis. Similar to MNV-1, Rg3 pretreatment did not impair EMCV replication in RAW cells (Supplementary Fig. S1G). However, a 3-day oral Rg3 administration prior to intraperitoneal (i.p.) EMCV injection led to a marked decrease in lethality (Fig. 1d) and alleviated neurovirulence compared with nontreated controls (Fig. 1e). This protective effect was abolished in Van-treated mice, and partially restored by FMT; there was a trend in lower lethality and neurovirulence for FMT plus Rg3 treatment versus FMT alone, although it was not statistically significant (Fig. 1d, e). In support of this, we observed that Van treatment vanished the inhibitory effect exerted by Rg3 on viral replication in the spleen and brain of EMCV-infected PBS mice, while microbiome reconstitution by FMT in Van-treated mice protected them from EMCV replication in these tissues in the presence of Rg3 (Fig. 1f). In summary, these data suggest that Rg3 can limit local and systemic enteric virus infections and ameliorate the resulting disease in a gut microbiome-dependent manner.
The protective effect of Rg3 against enteric virus infection results from enrichment of commensal Blautia spp.
Since Rg3 administration stimulated the growth of certain bacterial communities from the Blautia genus (Fig. 1b), we chose B. coccoides and B. obeum as representative isolates to determine how they protect against enteric virus infection. Van-treated mice were colonized with B. coccoides or B. obeum and then infected with MNV-1. Clostridium butyricum, which is a robust butyrate-producer that confers no protection against EMCV systemic infection [15], served as an unrelated Van-sensitive, Gram-positive control. As expected, colonization of Van-treated mice with B. coccoides or B. obeum markedly reduced the MNV-1 viral burden in the ileum, colon and MLNs whereas C. butyricum gavage failed to do so despite effective colonization (Fig. 1g and Supplementary Fig. S2A).
For EMCV protection assays, Van-treated mice were gavaged with B. coccoides, B. obeum or C. butyricum prior to i.p. EMCV inoculation. Survival rates increased from 10% in Van-treated mice to 50% and 60% in Van-treated animals colonized by B. coccoides or B. obeum, respectively, which was similar to the 40% survival rate of PBS control mice. C. butyricum did not alter EMCV lethality in Van-treated mice, despite effective colonization (Fig. 1h and Supplementary Fig. S2A). Moreover, Van-treated mice colonized with B. coccoides or B. obeum but not C. butyricum exhibited remarkably reduced viral titers in the brain and spleen compared with noncolonized controls (Supplementary Fig. S1I).
To further confirm a specific role for the commensal B. coccoides and B. obeum in modulating enteric virus infections, we repeated the colonization and EMCV infection studies in a germ-free (GF) mouse model. Following efficient colonization of GF mice (Supplementary Fig. S2B), significantly lower EMCV titers were detected at 3 dpi in the brain and spleen of mice colonized with B. coccoides or B. obeum but not C. butyricum (Fig. 1i). These concordant results in Van-treated and GF mice indicate that Blautia spp. can specifically modulate enteric virus infection both at mucosal and systemic sites.
Blautia-associated protection against enteric virus infection results from increased acetate and propionate concentrations
Given the significant increase in relative abundance of SCFA-producing bacteria in the mice administered Rg3 (Fig. 1b), we wanted to measure the changes in actual SCFA production. Using GC-MS to detect absolute SCFA concentrations in mouse fecal samples, we found a significant enhancement of SCFA after 3 days of oral Rg3 administration. This enhancement was abolished by Van treatment and partially reconstituted by FMT (Fig. 2a). Concentrations of acetate and propionate, but not butyrate, positively correlated with Blautia abundance (Fig. 2b), and negatively correlated with intestinal MNV-1 titers or splenic EMCV viral burden (Fig. 2c and Supplementary Fig. S3B).
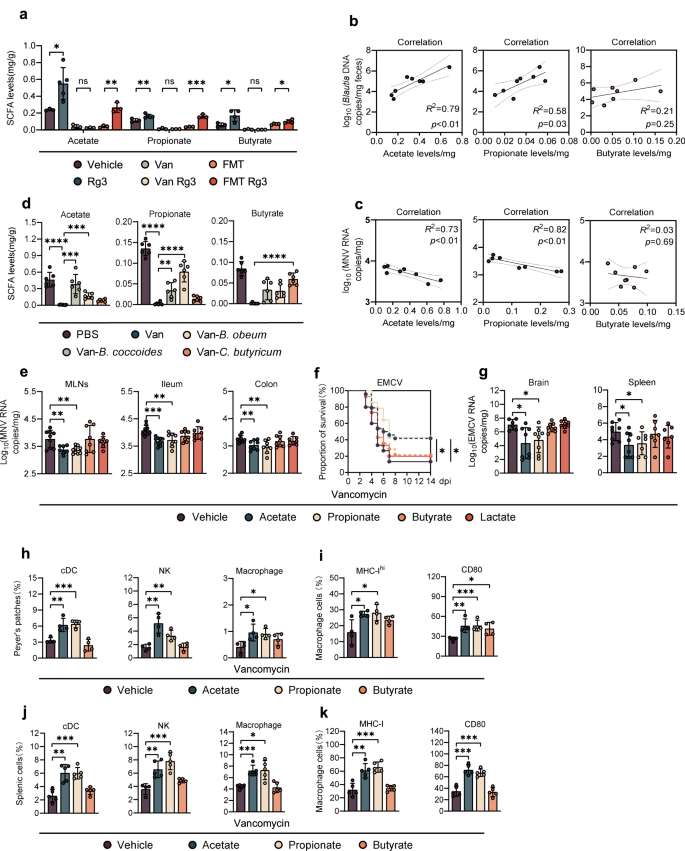
a Fecal SCFA concentration of vehicle-, Rg3-, Van-, Van-Rg3-, FMT- treated or FMT-Rg3-treated, uninfected mice (n = 3–4). b Correlation between relative fecal abundance of Blautia spp. and acetate (left), propionate (mid), or butyrate (right) concentration in Van-treated, Blautia-colonized mice (n = 8). c Correlation between fecal acetate (left), propionate (mid), butyrate (right) concentration and MNV viral burden in the ileum of Van-treated, Blautia-colonized mice (n = 8). d Fecal acetate (left), propionate (mid) and butyrate (right) concentration of Van-treated and Blautia- or C. butyricum-colonized, uninfected mice (n = 5–6). e Viral burden analysis at 1 dpi of MLNs, ileum and colon tissues collected from mice that were pretreated for 2 weeks with 200 mM acetate, propionate or butyrate in drinking water prior to oral infection with 2 × 107 TCID50 MNV (n = 8). f Survival curves of groups of Van-treated mice pretreated with 200 mM acetate, propionate or butyrate prior to i.p. infection with 2 × 102 TCID50 of EMCV (n = 13–24). g Viral titers at 3 dpi in brain and spleen collected from Van-treated mice pretreated with 200 mM acetate, propionate or butyrate prior to i.p. infection with 1 × 103 TCID50 of EMCV (n = 6–8). h–k Frequency of a panel of innate immune cells including conventional dendritic cells (cDCs), NK cells and macrophages from (h) the distal ileum PPs of MNV-1-infected mice at 1 dpi or (j) spleen of EMCV-infected mice at 3 dpi. Expression of MHC-I and CD80 on (i) PP macrophages from differently treated and MNV-infected mice at 1 dpi, or (k) splenic macrophages from differently treated and EMCV-infected mice at 3 dpi (n = 5–6). Plotted data represent the mean ± s.d. R2 and exact two-sided p values calculated by Pearson's test are shown. Unpaired two-tailed Student's t tests were used for statistical purposes. *p < 0.05; **p < 0.01; ***p < 0.001, ****p < 0.0001.
To further confirm the relationship between Blautia spp. and increased acetate/propionate production, we colonized Van-treated mice with B. coccoides, B. obeum or C. butyricum and quantified fecal SCFAs at 48 h post-colonization. B. coccoides, B. obeum and C. butyricum mostly restored the fecal concentration of acetate, propionate or butyrate, respectively, and partially rescued production of other SCFAs (Fig. 2d and Supplementary Fig. S3A). Consistently, we analyzed bacterial cultures of B. coccoides, B. obeum and C. butyricum with GC-MS and discovered a similar SCFA production to that observed in the in vivo colonization assay (Supplementary Fig. S3C).
To corroborate the protective role of SCFAs against local enteric virus infection, we directly administered acetate, propionate or butyrate in the drinking water of Van-treated mice for 2 weeks prior to enteric virus infection. Lactate was used as an unrelated glucose metabolite control. Fecal SCFA concentrations were significantly higher in the treated groups compared with the nontreated controls (Supplementary Fig. S3D); a significant reduction in intestinal and lymphatic MNV-1 burden was observed in mice given acetate or propionate, but not butyrate or lactate (Fig. 2e). In the more severe systemic EMCV infection model, acetate/propionate pretreatment reduced mortality by more than 20% (Fig. 2f), viral titers in the brain and spleen were remarkably decreased (Fig. 2g), and neuropathology was significantly ameliorated (Supplementary Fig. S3E). In contrast, butyrate or lactate treatment did not protect against EMCV based on survival curves, tissue viral burden or cerebral lesions (Fig. 2e, f, Supplementary Fig. S3E). Wild-type (WT) control mice treated with acetate or propionate had lower EMCV-induced lethality, and butyrate treatment even exacerbated mortality (Supplementary Fig. S3F).
SCFA-related protection from enteric virus infection is dependent on macrophages
To identify the cell types that participate in the early control of MNV-1 infection mediated by SCFAs, we first evaluated the recruitment and activation of early responding innate immune cells in the distal ileum Peyer's patches (PPs) at 1 dpi of MNV-1 infection by flow cytometry (Supplementary Fig. S4A). We observed a significant increase in the percentage of NK cells, macrophages, and conventional dendritic cells (cDCs) in Van-treated mice given acetate or propionate, but not butyrate compared with the PBS-treated control mice (Fig. 2h). In contrast, neither Van nor SCFA treatment altered the frequencies of T-cells or B-cells in the context of MNV-1 infection (Supplementary Fig. S4C). Furthermore, we discovered that pretreatment with acetate or propionate but not butyrate significantly augmented surface molecules associated with macrophage activation, such as MHC-I and CD80, upon MNV-1 infection (Fig. 2i).
Three days after EMCV infection, proportions of NK cells, macrophages and cDCs were greatly increased in the spleen of Van-treated mice given acetate or propionate but not butyrate prior to infection, compared with the PBS-treated controls (Fig. 2j and Supplementary Fig. S4B). Similar to MNV-1 infection, frequencies of T/B lymphocytes were not altered in the SCFA-treated mice (Supplementary Fig. S4D). MHC-I and CD80 were also upregulated 3 days after EMCV infection in the splenic macrophages from Van-treated mice given acetate or propionate but not butyrate (Fig. 2k).
To further determine the role of mononuclear phagocytes in SCFA-related protection against acute enteric virus infection, we depleted mononuclear phagocytes in Van-treated mice using clodronate liposomes and analyzed survival after EMCV inoculation. Clodronate greatly impaired the protection mediated by acetate and propionate treatment, suggesting that acetate/propionate-driven antiviral effect might be correlated to the activation of mononuclear phagocytes (Fig. 2f and Supplementary Fig. S4E). Furthermore, the protective patterns of acetate and propionate treatment were comparable between Van-treated WT B6 and Rag1−/− mice (lacking all T and B cell responses), suggesting that lymphocytes and adaptive immunity are dispensable for SCFA-driven protection against EMCV systemic infection (Supplementary Fig. S4F).
Rg3- and Blautia-derived acetate and propionate restrict enteric virus infection by stimulating IFN-I responses in macrophages
To determine whether acetate and propionate have a direct antiviral effect on macrophages, BMDMs were treated with SCFAs at different concentrations for 24 h before MNV-1 infection. Indeed, acetate or propionate pretreatment before MNV-1 infection significantly impaired viral replication in BMDMs in a dose-dependent manner, whereas butyrate did not affect MNV-1 growth whatsoever (Fig. 3a and Supplementary Fig. S5A). A similar protective pattern was observed in RAW264.7 cells treated with acetate or propionate prior to EMCV infection (Supplementary Fig. S5B).
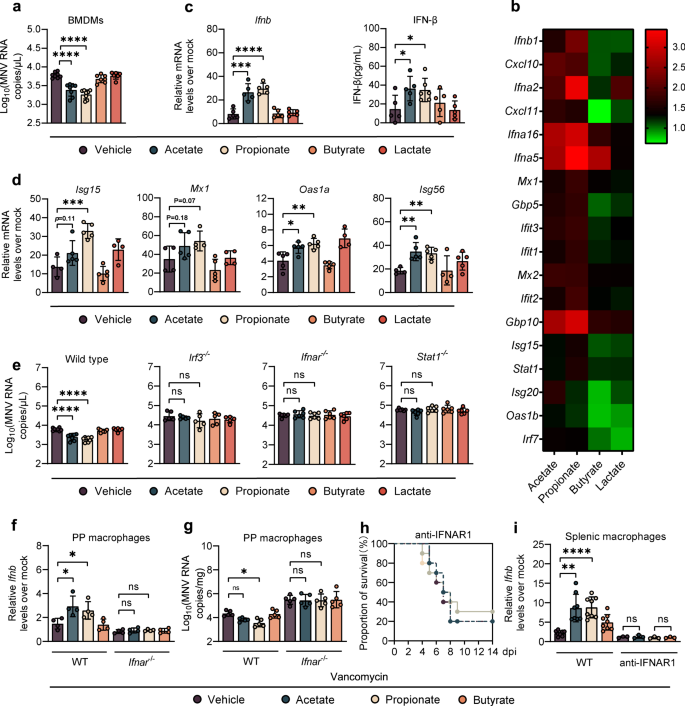
a Viral titers at 1 dpi in BMDMs from WT mice pretreated with 1 mM acetate, propionate, butyrate or 10 mM lactate for 24 h prior to MNV infection (MOI = 1) (n = 6–8). b Heatmap profiling the expression of 18 genes involved in IFN-β and ISGs. c Relative Ifnb expression (left, fold change compared with mock-infected BMDMs) and IFN-β protein (right) determined at 8 hpi in SCFA-treated BMDMs after MNV infection (n = 5–6). d Relative expression of different ISGs at 8 hpi in BMDMs pretreated for 24 h with 1 mM acetate, propionate, butyrate, or 10 mM lactate prior to MNV infection (n = 4–5). e Viral titers at 1 dpi in BMDMs collected from WT, Irf3−/−, Ifnar−/−, or Stat1−/− mice pretreated with 1 mM acetate, propionate, butyrate, or 10 mM lactate prior to MNV infection (n = 5–6). Relative Ifnb expression (f) and viral burden (g) at 1 dpi in PP macrophages sorted from Van- and SCFA-pretreated, MNV-infected WT or Ifnar−/− mice (n = 4–5). h Survival curves for groups of Van- and SCFA-pretreated mice treated with anti-IFNAR1 IgG prior to i.p. infection with EMCV (n = 8). i Relative Ifnb expression in splenic macrophages at 3 dpi sorted from Van- and SCFA-pretreated, EMCV-infected WT or anti-IFNAR1 IgG treated mice (n = 5). Plotted data represent the mean ± s.d. Unpaired two-tailed Student's t tests were used for comparison of means between treatment groups. *p < 0.05; **p < 0.01; ***p < 0.001; ****p < 0.0001; ns not significant.
To define how acetate and propionate mediate antiviral macrophage functions against MNV-1, we performed transcriptional profiling of infected BMDMs with or without acetate, propionate, butyrate or lactate pretreatment. At 8 h post-infection (hpi), unbiased hierarchical clustering and GO analyses indicated that IFN-I signaling was the most significantly enriched pathway in acetate- and propionate-pretreated BMDMs compared with butyrate-, lactate- or vehicle-pretreated cells (Fig. 3b and Supplementary Fig. S5C). Consistent with this, pretreatment with acetate or propionate but not butyrate or lactate led to significantly higher IFN-β expression in BMDMs, at both the transcriptional and translational level (Fig. 3c). However, there was no apparent IFN-β upregulation in uninfected BMDMs pretreated with acetate or propionate (Supplementary Fig. S5D). These data, concordant with the result of transcriptome analysis, suggest that the antiviral, but not basal, transcriptional IFN-I response program of macrophages is altered by acetate and propionate. We also evaluated the expression of proinflammatory cytokines including IL-1β, IL-6 and TNF-α using qRT-PCR and found increased levels of cytokine transcripts in infected BMDMs pretreated with acetate or propionate compared with those receiving butyrate, lactate or untreated cells (Supplementary Fig. S5E), indicating that acetate and propionate pretreatment caused a generally enhanced innate antiviral immune response against MNV-1 infection.
Consistent with the IFN-β analysis, there was a marked upregulation of IFN-stimulated genes (ISGs) including Isg15, Isg56, Oas1a and Mx1 in the BMDMs pretreated with acetate or propionate compared with those receiving butyrate or lactate (Fig. 3d). Further, EMCV-stimulated BMDMs exhibited a significant increase in IFN-β expression in the presence of acetate or propionate (Supplementary Fig. S5F). We also measured MNV-1 replication in BMDMs isolated from Irf3−/−, Ifnar−/− and Stat1−/− mice and observed no differences in viral titers regardless of SCFA treatment (Fig. 3e), demonstrating that inhibition of MNV-1 by acetate and propionate depends on important signaling molecules in the IFN-I induction pathway and amplification loop.
We sought to confirm the role of acetate and propionate in enhancing IFN-I production and restricting MNV-1 infection in macrophages at mucosal sites and found increased expression of IFN-β and decreased viral titers in the PP macrophages of MNV-1-infected, Van-treated mice administrated with acetate or propionate compared to vehicle- or butyrate-treated controls at 1 dpi (Fig. 3f, g). However, the effects of acetate or propionate on enhancement of IFN-β expression and reduction of MNV-1 replication were diminished in Van-treated Ifnar−/− mice (Fig. 3f, g). In support of this finding, we observed that Rg3 administration increased IFN-β expression in the PPs of MNV-1-infected WT B6 mice, while Van treatment eliminated this phenotype (Supplementary Fig. S6A, B). Correspondingly, colonization of B. coccoides or B. obeum reestablished IFN-I responses and control of viral replication in macrophages isolated from PPs of MNV-1 infected, Van-treated WT B6 mice at 1 dpi, whereas the effects of Blautia colonization were diminished in Van-treated Ifnar−/− mice (Supplementary Fig. S6C, D).
Since EMCV infection overwhelms the defenses of Ifnar−/− mice [15], we injected Van-treated WT B6 mice with an anti-IFNAR1 antibody [18] to evaluate the role of IFN-I signaling in acetate/propionate-driven protection against systemic EMCV infection. Protection against lethal systemic EMCV infection conferred by acetate/propionate supplementation (Fig. 2f) in Van-treated mice was diminished when these animals were administrated with the IFNAR1-blocking antibody (Fig. 3h). Acetate/propionate supplementation also caused elevated expression of IFN-β in splenic macrophages of EMCV-infected, Van-treated WT B6 mice at 3 dpi, but failed to do so in Van-treated mice administrated with the IFNAR1-blocking antibody (Fig. 3i). Supporting these data, we observed no differences in mortality rate between IFNAR1-blocked mice regardless of Rg3 treatment, whereas WT mice given a nonspecific control IgG showed a significantly higher survival rate after treatment with Rg3 compared with vehicle control (Supplementary Fig. S6E). Moreover, elevated expression of IFN-β was exhibited in splenic macrophages of Rg3- versus vehicle-treated WT B6 mice following EMCV infection, an effect that was neutralized by Van pretreatment (Supplementary Fig. S6F).
Consistently, the protective effect exerted by B. coccoides or B. obeum colonization (Fig. 1d) was abolished in Van-treated mice administered the IFNAR1-blocking antibody (Supplementary Fig. S6G). Furthermore, IFN-β expression was restored in the splenic macrophages of EMCV-infected, Van-treated WT mice, but not those received IFNAR1-blocking antibody following Blautia spp. colonization at 3 dpi (Supplementary Fig. S6H). Taken together, these results indicate that acetate and propionate, which are increased after Rg3 administration and Blautia spp. colonization, restrict both local and systemic infection of enteric viruses in an IFN-I signaling-dependent manner.
Stimulation of IFN-I response in macrophages by acetate or propionate requires GPR43 signaling
SCFAs can exert their functions via binding to GPR43 and GPR41 [19], and it has been shown that GPR43 is essential for acetate-induced IFN-I responses in pulmonary epithelial cells during RSV infection [9]. Considering this, we repeated the in vitro experiments in Fig. 3a using a GPR43 agonist (4-CMTB) or a GPR41 agonist (AR420626). We found that 4-CMTB, but not AR420626, caused the same pattern of response observed with acetate or propionate in MNV-1-infected BMDMs, reflected by reduced virus load and upregulated IFN-β (Fig. 4a, b). Additionally, 4-CMTB protected the WT B6 mice against MNV-1 infection by reducing the viral burden and augmenting IFN-β in PP macrophages at 1 dpi (Fig. 4c, d).
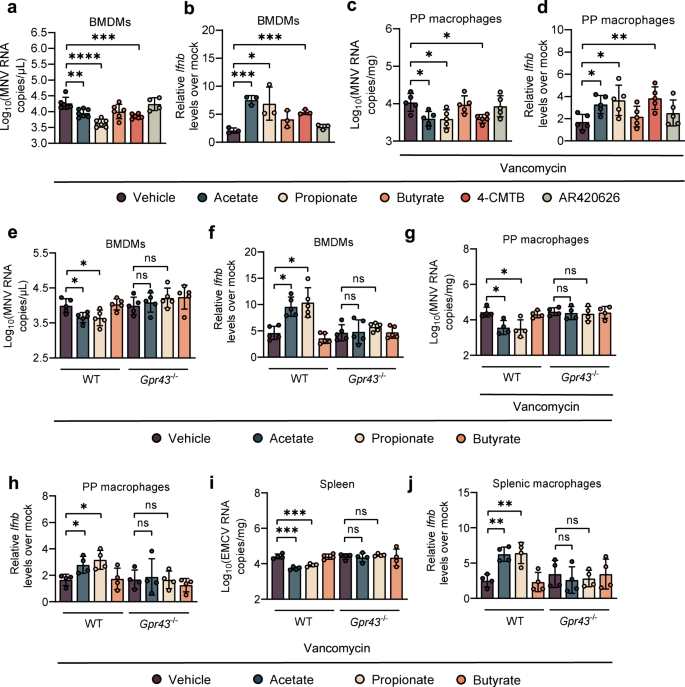
Viral titers (a) at 24 hpi and relative Ifnb expression (b) at 8 hpi in WT BMDMs pretreated with acetate, propionate, butyrate, or GPR43 and GPR41 agonists (4-CMTB and AR420626) and subsequently infected with MNV (a, n = 6; b, n = 3). Viral titers (c) and relative Ifnb expression (d) at 1 dpi in PP macrophages sorted from Van- and SCFA-, 4-CMTB- or AR420626-pretreated, MNV-infected mice (n = 5). Viral titers (e) at 24 hpi and relative Ifnb expression (f) at 8 hpi in Van- and SCFA-pretreated, MNV-infected BMDMs (n = 5). Viral titers (g) and relative Ifnb expression (h) at 1 dpi in PP macrophages sorted from Van- and SCFA-pretreated, MNV-infected WT or Gpr43−/− mice (n = 4). Viral titers (i) in splenocytes and Ifnb levels (j) in splenic macrophages collected at 3 dpi from Van- and SCFA-treated, EMCV-infected WT or Gpr43−/− mice (n = 4). Plotted data represent the mean ± s.d. Unpaired two-tailed Student's t tests were used for comparison of means between treatment groups. *p < 0.05; **p < 0.01; ***p < 0.001; ****p < 0.0001; ns not significant.
To elucidate the dependence of acetate/propionate stimulation of antiviral IFN-I on one of these receptors, we extracted BMDMs from WT B6 and GPR43 knockout (Gpr43−/−) mice, followed by acetate/propionate treatment and MNV-1 infection. Acetate/propionate pretreatment failed to reduce viral load or augment IFN-β expression in MNV-1-infected BMDMs extracted from Gpr43−/− mice compared with WT B6 mice (Fig. 4e, f). Butyrate treatment had no effect on MNV-1 replication or IFN-I responses in BMDMs regardless of genotype (Fig. 4a–d). Similarly, siRNA knockdown of Gpr43, but not Gpr41 significantly impaired MNV-1 replication and augmented IFN-β expression by the acetate/propionate treatment in WT B6 BMDMs (Supplementary Fig. S7A–C).
To further investigate the role of GPR43 in acetate/propionate-induced IFN-I responses against MNV-1 infection in vivo, Van-treated WT and Gpr43−/− mice were administrated with acetate or propionate and then inoculated with MNV-1. No differences in viral titers and IFN-β levels were seen in PP macrophages from MNV-1-infected, Van-treated Gpr43−/− mice, regardless of SCFA treatment (Fig. 4g, h).
Moreover, EMCV stimulation of acetate/propionate-pretreated BMDMs isolated from Gpr43−/− mice failed to upregulate IFN-β expression compared with WT B6 control BMDMs (Supplementary Fig. S7D). Similar to the results of MNV-1 in vivo study, there were no differences between the acetate/propionate-pretreated and control groups in Gpr43−/− mice with respect to viral burden in the spleen or IFN-β expression in the splenic macrophages, reflecting abrogated antiviral effect-induced by acetate/propionate in the absence of GPR43 (Fig. 4i, j). Thus, specific interaction between acetate/propionate and GPR43-primed macrophage IFN-I responses to prevent both local and systemic enteric virus infection.
Acetate and propionate promote antiviral IFN-I response by upregulating intracellular calcium influx and virus-induced mtDNA release in macrophages depending on GPR43 and MAVS
Acetate stimulates GPR43 and induces intracellular calcium influx in myotube cells [20] and it has been shown that IFN-I response is connected to the intracellular Ca2+ levels and can be suppressed in the presence of Ca2+ chelators [21]. Thus, we pretreated BMDMs isolated from WT B6 mice with SCFAs and 4-CMTB. As predicted, intracellular Ca2+ levels were significantly induced by acetate, propionate, or 4-CMTB, but not by butyrate treatment (Fig. 5a, b). However, this effect was completely abolished in BMDMs collected from Gpr43−/− mice (Supplementary Fig. S8A). WT BMDMs primed by acetate/propionate had significantly lower IFN-β levels and higher MNV-1 titers when cultured with Ca2+-free cell culture medium, an outcome reversed by addition of Ca2+ (Fig. 5c, d). Similarly, treatment of WT BMDMs with a cell-permeable Ca2+ chelator BAPTA-AM prevented the IFN-β upregulation and MNV-1 inhibition resulting from acetate/propionate treatment (Supplementary Fig. S8B, C). Thus, enhanced IFN-I production and MNV-1 inhibition was mediated by intracellular calcium influx in macrophages, which was modulated by GPR43 signaling.
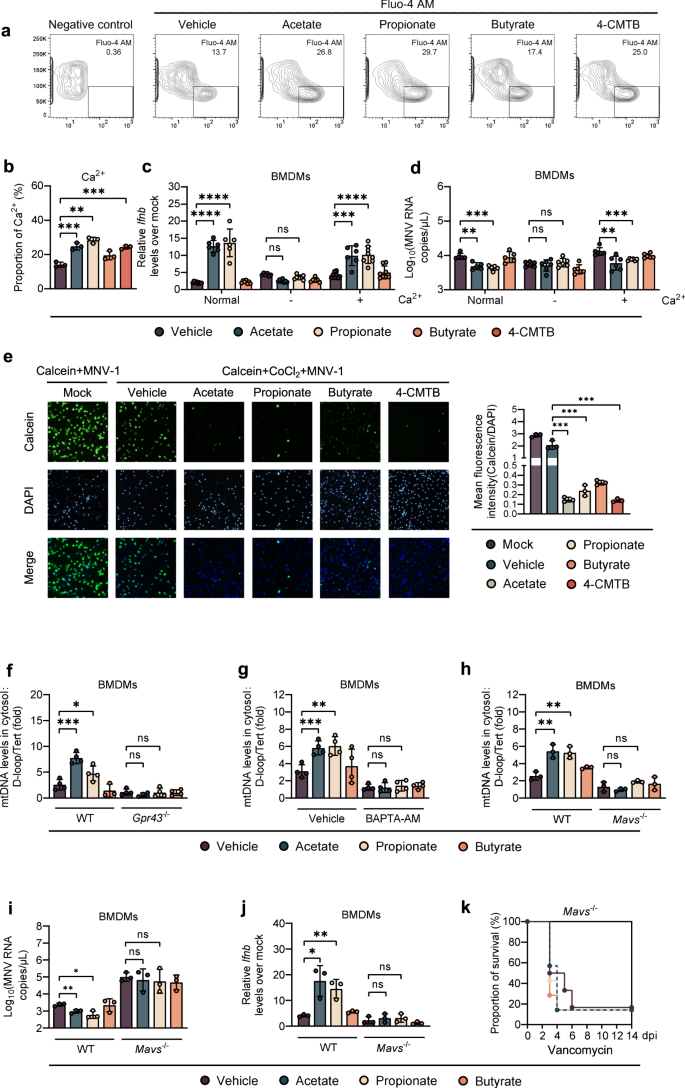
a, b Intracellular Ca2+ levels in WT BMDMs were measured after 24 h incubation with acetate, propionate, butyrate or 4-CMTB. c, d BMDMs were cultured in normal medium or calcium-free medium with or without supplementation with 0.2 g/L Ca2+. Relative Ifnb expression (c) at 8 hpi or viral titers (d) at 24 hpi in SCFA-pretreated, MNV-infected WT BMDMs were analyzed (n = 6). e The openness of mitochondrial permeability transition pore (mPTP) was determined at 24 hpi by mPTP kit in SCFA- and 4-CMTB-pretreated, MNV-infected BMDMs. f SCFA-pretreated WT or Gpr43−/− BMDMs were infected with MNV for 24 h and cytosolic mtDNA was assessed by quantitative PCR (n = 4). g WT BMDMs were treated with 30 µM BAPTA-AM for 24 h, followed by 24 h incubation with SCFAs prior to MNV infection. Cytosolic mtDNA levels were analyzed at 24 hpi (n = 4). h Cytosolic mtDNA levels were measured at 24 hpi in WT or Mavs−/− BMDMs pretreated with acetate, propionate, or butyrate and infected with MNV (n = 3). Viral titers (i) at 24 hpi and Ifnb levels (j) at 8 hpi in SCFA-pretreated, MNV-infected WT or Mavs−/− BMDMs (n = 3). k Groups of differently treated Mavs−/− mice were i.p. infected with EMCV, and survival curves were documented (n = 6–7). Plotted data represent the mean ± s.d. Unpaired two-tailed Student's t tests were used for comparison of means between treatment groups. *p < 0.05; **p < 0.01; ***p < 0.001; ****p < 0.0001, ns not significant.
Changes in cytosolic Ca2+ causes a mitochondrial permeability transition, which leads to the leakage of mtDNA into the cytoplasm [22]. Thus, we hypothesized that changes of intracellular calcium influx caused by GPR43 activation enhance mtDNA leakage after enteric virus infection. To test this, we first evaluated the degree of mitochondrial permeability transition pore (mPTP) opening using a specific kit. We observed a significantly higher degree of mPTP opening (dimmer green fluorescence) in acetate-, propionate- or 4-CMTB-treated BMDMs compared to vehicle- or butyrate-treated BMDMs upon MNV-1 infection (Fig. 5e). However, this effect was diminished by treatment with BAPTA-AM (Supplementary Fig. S8D). Consistently, we found that cytosolic mtDNA release was triggered by MNV-1 infection in WT BMDMs (3-fold higher compared to uninfected cells), and was significantly enhanced by pretreatment with acetate or propionate but not butyrate; this effect was completely abrogated by GPR43 depletion or BAPTA-AM treatment (Fig. 5f, g).
As it has been reported that mtDNA release during IAV infection requires activation of MAVS-dependent signals [23], we next examined whether MAVS is required for acetate/propionate-enhanced mtDNA release triggered by MNV-1 infection. To this end, we infected WT or MAVS-deficient BMDMs with MNV-1 following acetate/propionate treatment. Indeed, we found that the acetate/propionate-enhanced, MNV-1 induced mtDNA release into the cytosol was abolished in BMDMs isolated from Mavs−/− mice (Fig. 5h). In addition, Acetate/propionate pretreatment did not lead to descending viral load or ascending IFN-β expression in MNV-1-infected, MAVS-deficient BMDMs (Fig. 5i, j). Moreover, Blautia spp. colonization or acetate/propionate treatment in Van-treated Mavs−/− mice conferred no protection against systemic EMCV infection (Fig. 5k and Supplementary Fig. S8E).
Acetate and propionate enhance IFN-I response in macrophages and limit enteric virus infection through the GPR43-cGAS-STING signaling axis
The importance of cytosolic mtDNA in guanosine monophosphate-adenosine monophosphate synthase (cGAS)- and stimulator of IFN genes (STING)-mediated IFN-I expression and innate immune defense against RNA viruses has been highlighted recently [23,24,25]. To this end, we then tested whether the acetate/propionate-induced intracellular calcium influx triggered by GPR43 activation activated cGAS in MNV-1-infected BMDMs. As expected, acetate/propionate stimulation showed no effect on enhancement of cGAS expression upon MNV-1 infection when the WT BMDMs were cultured in Ca2+-free cell culture medium, while Ca2+ supplementation reestablished this effect (Fig. 6a). A similar pattern of reduction of cGAS expression was observed when BAPTA-AM was used on WT BMDMs (Supplementary Fig. S8F). Consistently, the acetate/propionate-induced increase in cGAS expression after MNV-1 infection was dependent on GPR43 (Fig. 6b and Supplementary Fig. S8G). Moreover, acetate/propionate pretreatment did not affect viral replication or IFN-β expression in BMDMs treated with cGAS or STING siRNA or in BMDMs isolated from Sting−/− mice (Fig. 6c, d and Supplementary Fig. S8H, I). Furthermore, we demonstrated that pretreatment with acetate or propionate but not butyrate markedly increased the level of cGAS, phosphorylated STING and IRF3 upon MNV-1 infection, differences that were lost in conditions of Ca2+ or GPR43 depletion (Fig. 6e).
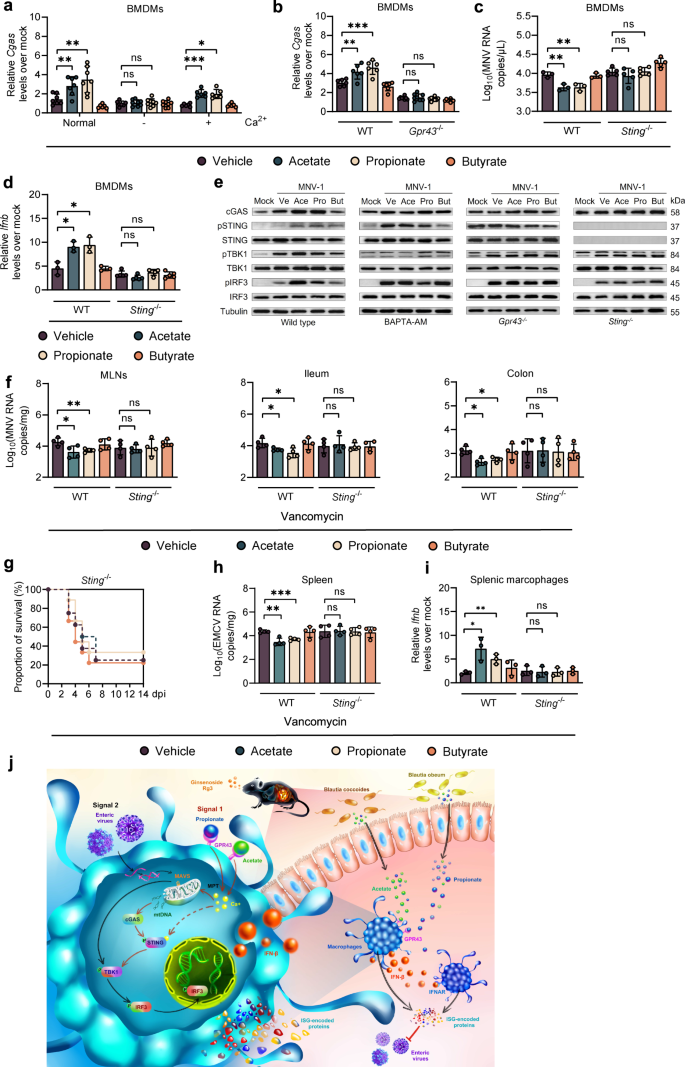
a Relative Cgas expression at 8 hpi in WT BMDMs cultured in normal medium or calcium-free medium with or without supplementation with 0.2 g/L Ca2+ pretreated with SCFAs and infected with MNV. b Relative Cgas expression at 8 hpi in BMDMs from WT or Gpr43−/− mice pretreated with SCFAs and infected with MNV. Viral titers (c) at 24 hpi and ifnb levels (d) at 8 hpi in SCFA-pretreated, MNV-infected WT or Sting−/− BMDMs (n = 3–5). e Western blot of cGAS, IRF3, pIRF3, STING, pSTING, TBK1, pTBK1 and tubulin in WT BMDMs with or without 30 µM BAPTA-AM, or BMDMs from Gpr43−/− or Sting−/− mice. f Viral burden analysis at 1 dpi...
Comments
Post a Comment