Airway epithelial CD47 plays a critical role in inducing influenza virus-mediated bacterial super-infection - Nature.com
Abstract
Respiratory viral infection increases host susceptibility to secondary bacterial infections, yet the precise dynamics within airway epithelia remain elusive. Here, we elucidate the pivotal role of CD47 in the airway epithelium during bacterial super-infection. We demonstrated that upon influenza virus infection, CD47 expression was upregulated and localized on the apical surface of ciliated cells within primary human nasal or bronchial epithelial cells. This induced CD47 exposure provided attachment sites for Staphylococcus aureus, thereby compromising the epithelial barrier integrity. Through bacterial adhesion assays and in vitro pull-down assays, we identified fibronectin-binding proteins (FnBP) of S. aureus as a key component that binds to CD47. Furthermore, we found that ciliated cell-specific CD47 deficiency or neutralizing antibody-mediated CD47 inactivation enhanced in vivo survival rates. These findings suggest that interfering with the interaction between airway epithelial CD47 and pathogenic bacterial FnBP holds promise for alleviating the adverse effects of super-infection.
Similar content being viewed by others
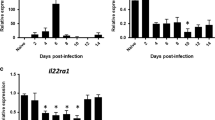
IL-22-binding protein exacerbates influenza, bacterial super-infection
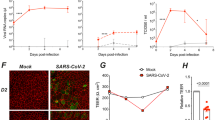
SARS-CoV-2 infection induces the dedifferentiation of multiciliated cells and impairs mucociliary clearance
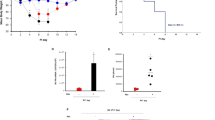
Nasal symbiont Staphylococcus epidermidis restricts the cellular entry of influenza virus into the nasal epithelium
Introduction
The 1918 influenza (H1N1) pandemic claimed the lives of more than 50 million people worldwide1. Most deaths were due to secondary bacterial pneumonia caused by common upper respiratory tract bacteria, such as Staphylococcus aureus and Streptococcus pneumoniae. Similarly, the illness of patients in the intensive care unit, infected with 2009 influenza A (H1N1), was exacerbated by secondary bacterial infections, with high morbidity and mortality rates2,3. The development and extensive use of antibiotics have played a crucial role in reducing the severity of secondary bacterial infections. However, the recent emergence of antibiotic resistance has introduced a significant level of complexity to the treatment of these secondary bacterial infections4. Consequently, there is an urgent need to investigate and uncover the elusive molecular mechanisms responsible for secondary bacterial infections triggered by influenza.
Airway epithelial cells establish apical junctional complexes between neighboring cells to serve as a protective barrier against the external environment. Apical junctional complexes consist of apical tight junctions and underlying adherens junctions, which facilitate cell–cell adhesion and maintain barrier integrity. Zonula occludens (ZO, e.g., ZO-1) and catenin (e.g., β-catenin) proteins connect the intracellular domains of tight junctions (e.g., Claudins and Occludin) and adherens junctions (e.g., E-cadherin) with cytoskeletal components, forming "cytosolic plaques"5,6. Tight junctions play a critical role in regulating the passage of ions and solutes through the paracellular space, effectively blocking the translocation of pathogens from the lumen to the interstitium. Consequently, viral infections that disrupt tight junctions can facilitate the translocation of pathogens and receptor exposure7,8,9,10. Thus, preserving the integrity of this barrier is crucial in preventing bacterial infection.
Respiratory viral infection increases host susceptibility to bacterial pathogens by i) interfering with antibacterial innate immune responses via interferon (IFN) induction11,12,13,14,15,16,17 and depletion of alveolar macrophages18; or ii) providing binding sites for bacteria, including cellular receptors, such as intercellular adhesion molecule-1 (ICAM-1)19,20,21,22,23, carcinogenic embryonic adhesion molecule 1 (CEACAM1)20,24, platelet-activating factor receptor (PAF-r)20,24, or extracellular matrix (ECM) proteins, such as fibronectin (FN)24,25. Given that PAF-r binds to the phosphorylcholine on S. pneumoniae's cell wall, it has been proposed as a potential therapeutic target for secondary bacterial infections26,27. However, the use of a PAF-r antagonist did not demonstrate any effect in the secondary bacterial infection model28. As a result, it is essential to explore intervention strategies that specifically focus on disrupting the interaction between bacteria and cell receptors, utilizing antibody-mediated blockade, in treatment approaches.
CD47, also known as integrin-associated protein (IAP), is a widely expressed transmembrane glycoprotein. It serves as a "don't-eat-me" signal by interacting with the inhibitory receptor signal-regulatory protein alpha (SIRPα) on myeloid immune cells, thereby inhibiting phagocytosis of CD47-expressing erythrocyte29. Cancer and viral-infected hematopoietic cells overexpress CD47 for immune evasion30,31. Meanwhile, in non-hematopoietic cells, CD47 plays a role in tissue repair, contributing to improved healing and survival in various models such as skin thermal injury32, organ transplant33,34,35,36,37, and intestinal mucosal injury38. Notably, the specific involvement of CD47 in airway epithelium during super-infection remains unexplored. In a proteomics analysis of nasal epithelial cells infected with influenza virus, we observed the presence of CD47. Through the validation process, we uncovered that CD47 induced by viral infection was not only detected in the colonization site (nasal epithelium) but also at the site of infection (bronchial epithelium). Given that CD47 seems to be predominantly expressed in FoxJ1+ cells (deuterosomal cells and, to a lesser extent, multi-ciliated cells) based on single-cell RNA sequencing and immunostaining analysis of upper and lower airway primary epithelial cells, we initiated an investigation into the involvement of CD47 in secondary bacterial infections using a FoxJ1+ cell-specific CD47 gene-deletion mouse model and CD47 neutralizing antibodies. In our pursuit to identify a bacterial component that interact with CD47, we conducted experiments involving five mutant strains of S. aureus with deleted cell wall-anchored proteins. Remarkably, our investigations revealed that only fibronectin-binding proteins (FnBP) exhibited a strong affinity for CD47. To further solidify our findings, we employed a combination of FoxJ1+ cell-specific CD47 gene-deletion mice and a FnBP mutant strain of S. aureus in the context of super-infection. This approach allowed us to establish that the specific interaction between airway epithelial CD47 and bacterial FnBP plays a pivotal role in causing super-infection. By comprehensively exploring the role of CD47 in facilitating secondary bacterial infections, this study may pave the way for the development of innovative therapeutic approaches, ultimately leading to improved clinical outcomes for patients.
Results
CD47 is upregulated and localized to the apical surface of ciliated cells following infection of HBECs and HNECs with influenza virus
Limited research has investigated the localized surface remodeling of primary human nasal epithelial cells (HNECs) in the context of viral infection10,23,24,39. Consequently, the complete spectrum of upregulated adhesion molecules during respiratory viral infections that facilitate bacterial attachment and invasion remains unknown. To address this gap, the present study provides a comprehensive examination of HNEC responses to influenza virus pH1N1 infection. This investigation employs global proteome profiling of uninfected (Mock, n = 4) and influenza-infected (Virus-only, n = 4) HNECs utilizing isobaric tags for relative and absolute quantitation (iTRAQ) technique. A total of 3583 proteins were detected, 89 of which were significantly increased (52 proteins) or decreased (37 proteins) in the virus-infected HNECs compared to mock-infected controls (p < 0.05) (Supplementary Fig. 1a, b). Kyoto Encyclopedia of Genes and Genomes (KEGG) pathway analysis revealed four significantly enriched virus-related pathways in the virus-infected HNEC proteome, including "Influenza A" (Supplementary Fig. 1c). At three days post-infection (dpi), viral gene expression was elevated, accompanied by an increase in host innate immune responses, particularly type-I and type-III interferons (IFNs) (Supplementary Fig. 1d), while type-II IFN remained unchanged. Following viral infection, two candidate proteins displayed increased peptide expression and were expected to localize in the cellular membrane: leukocyte surface antigen CD47 (Q08722, FC = 1.15, p = 0.001), and carcinoembryonic antigen-related cell adhesion molecule 5 (CEACAM5, P06731, FC = 1.14, p = 0.028) (Supplementary Fig. 1b). Among these candidates, CD47 was selected for further investigation, and its expression was validated in both HNECs (Supplementary Fig. 2) and primary human bronchial epithelial cells (HBECs) (Fig. 1). Subsequent to viral infection, ZO-1 abundance decreased, and its cellular connections were disrupted, while CD47 exhibited increased expression in both HNECs (Supplementary Fig. 2a–c) and HBECs (Fig. 1a–c). In line with a prior study38, functional annotation using gene ontology (GO) analysis demonstrated that CD47's biological processes were linked to response to stimulus-related GOs, cell migration-related GOs, immune system process-related GOs, cell surface receptor signaling pathway, and cellular component organization (Supplementary Fig. 1e).
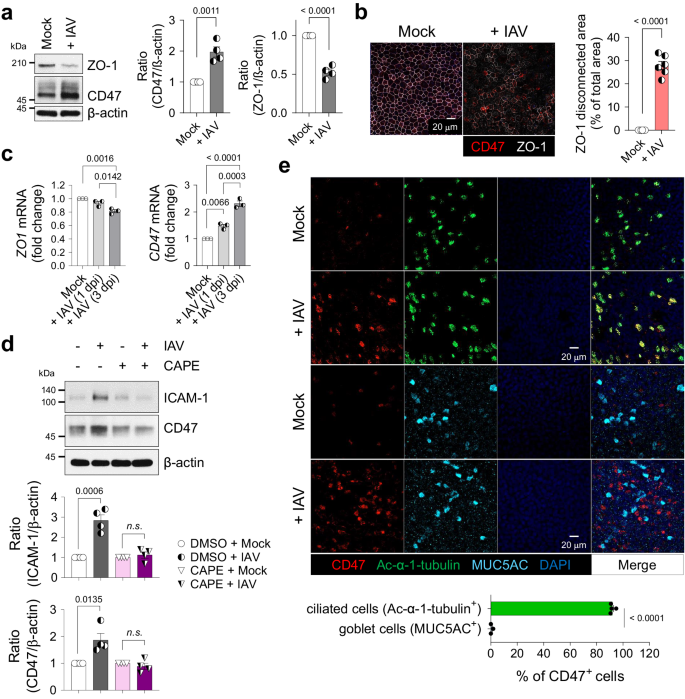
HBECs were infected with (+ IAV) or without (Mock) influenza A virus. a Immunoblot analysis of junction protein ZO-1 and surface protein CD47 at 1 day post-infection (dpi). Normalized CD47 and ZO-1 protein levels are presented as bar graphs (Mock, n = 4; + IAV, n = 4). b Representative whole-mount images of ZO-1 (white) and CD47 (red) at 1 dpi. The area where ZO-1 disconnection occurred are presented as bar graphs (Mock, n = 6; + IAV, n = 6). c Quantitative PCR (qPCR) analysis of ZO-1 and CD47 mRNAs at 1 and 3 dpi [Mock, n = 3; + IAV (1 dpi), n = 3; + IAV (3 dpi), n = 3]. d HBECs were treated with or without 10 μM NF-κB inhibitor caffeic acid phenethyl ester (CAPE) for 1 h before influenza virus infection. Immunoblot analysis of ICAM-1 and CD47 at 1 dpi. Normalized ICAM-1 and CD47 protein levels are presented as bar graphs (DMSO + Mock, n = 4; DMSO + IAV, n = 4; CAPE + Mock, n = 4; CAPE + IAV, n = 4). e Whole-mount images of influenza virus-infected HBECs. Co-staining of CD47 (red) and ciliated cell-specific marker protein Ac-α-tubulin (green, n = 4) or goblet cell-specific marker protein MUC5AC (cyan, n = 3). Percentages of CD47-positive cells are presented as bar graphs. Data are presented as mean values ± SEM. Significance was determined by unpaired two-tailed Student's t test or one-way ANOVA with Tukey's multiple comparisons test. n.s. not significant. Source data are provided as a Source Data file.
Given that CD47 is transcriptionally regulated by nuclear factor kappa B (NF-κB) in a hepatocellular carcinoma mouse model40, we examined whether airway epithelial CD47 is similarly regulated by the NF-κB pathway. In HBECs, we confirmed the activation of the NF-κB pathway induced by viral infection, evidenced by p65 phosphorylation at 1 h post-infection (hpi), along with the induction of the downstream target protein, intercellular adhesion molecule-1 (ICAM-1)19,23. CD47 protein abundance exhibited a slight increase after 1 hpi and reached its peak at 12 hpi (Supplementary Fig. 3a). Pre-treatment of HBECs with caffeic acid phenethyl ester (CAPE), a specific inhibitor of NF-κB, resulted in the inhibition of viral infection-induced p65 phosphorylation at 1 hpi (Supplementary Fig. 3b). Additionally, the upregulation of ICAM-1 and CD47 at 24 hpi was blocked (Fig. 1d), confirming the involvement of the NF-κB pathway in mediating CD47 upregulation in airway epithelial cells during viral infection. We also conducted an extensive investigation to determine whether CD47 induction is dependent on IFN, the production of which is NF-κB-dependent41. We observed an increase in CD47 expression following viral infection (Supplementary Fig. 3c, d), and this effect was replicated in HBECs treated with recombinant human IFN-β (Supplementary Fig. 3e) and IFN-λ1 (Supplementary Fig. 3f). Notably, the induction of CD47 by influenza virus was entirely suppressed when anti-IFNAR (Supplementary Fig. 3c) or anti-IFNLR (Supplementary Fig. 3d) neutralizing antibodies were applied. Additionally, the induction of CD47 by recombinant human IFN-β was effectively inhibited by anti-IFNAR neutralizing antibodies (Supplementary Fig. 3e), while CD47 induction by recombinant human IFN-λ1 was specifically blocked by anti-IFNLR neutralizing antibodies (Supplementary Fig. 3f). These results collectively demonstrate that CD47 induction occurs via the NF-κB/IFN pathway.
To ascertain if viral infection-induced CD47 expression is specific to certain cell types, we conducted co-immunostaining of airway epithelial cells and observed that CD47+ HNECs (Supplementary Fig. 2d) and CD47+ HBECs (Fig. 1e) were co-localized with the ciliated cell-specific marker, Ac-α-tubulin (92.0 ± 1.0% in HNECs; 91.1 ± 0.6% in HBECs) rather than the goblet cell-specific marker, MUC5AC (0.6 ± 0.6% in HNECs; 3.0 ± 1.6% in HBECs), during viral infection. Interestingly, co-immunostaining of CD47 and influenza nucleoprotein (NP) revealed that not all CD47-positive cells completely overlapped with viral protein (Supplementary Fig. 3g). It's worth noting that ciliated cells are a part of the epithelium, lining the apical surface of the respiratory tract and exposing their cilia to the lumen42. Therefore, the detection of CD47 co-expressed with Ac-α-tubulin indicates that it is present on the apical side of the ciliated cells. In a similar context, the analysis of HNEC single-cell RNA sequencing (scRNA-seq) data revealed that CD47+ clusters primarily consist of FOXJ1+ cells, with a notable prevalence of deuterosomal cells and a partial presence of multi-ciliated cells (Supplementary Fig. 2e)43. These results reinforce a fundamental aspect of this study, underscoring the specific presence of CD47 expression in ciliated epithelial cells. Fluorescence-activated cell sorting (FACS) analysis further confirmed the presence of CD47 protein on the cell surface of HNECs, with increased expression observed during viral infection (Supplementary Fig. 2f). Therefore, these findings demonstrate that CD47 is induced and exposed on the apical surface of ciliated cells in respiratory epithelial cells infected with the influenza virus.
Epithelial CD47 in HBECs and HNECs facilitates super-infection
To elucidate the mechanisms underlying susceptibility to secondary bacterial infection induced by viral infection, we developed an in vitro model of super-infection using HNECs and HBECs (Supplementary Fig. 4). HNECs simulate the upper airway, the initial site of viral infection, while HBECs represent the lower airway, where super-infection has a terminal impact. Following air–liquid interface (ALI) culture for 14 days (HNECs) or 21 days (HBECs), cells were infected with influenza virus pH1N1 and/or S. aureus. Since ZO-1 displayed reduced levels and disconnection during viral infection, we assessed the impact of secondary bacterial infection on HNECs and HBECs by evaluating paracellular permeability, changes in cellular morphology, and cell viability. When cells were co-infected with virus (MOI 1) and bacteria (MOI 5 for HNECs; MOI 3 for HBECs), paracellular permeability of fluorescein isothiocyanate (FITC)-dextran was significantly elevated (Supplementary Fig. 4a, e), and trans-epithelial electrical resistance (TEER) showed a substantial decrease (Supplementary Fig. 4b, f), as compared to mock-infected HNECs and HBECs. Notably, both of these parameters remained unchanged in cells subjected to either virus or bacteria single infection. Although no significant detrimental effect of super-infection was observed in lactate dehydrogenase (LDH) assay at 3 dpi (Supplementary Fig. 4c, g), visible cytopathogenic effects resulting from super-infection became apparent after 7 days in HNECs and 5 days in HBECs (Supplementary Fig. 4d, h). Given that HNECs exhibited greater resistance to super-infection compared to HBECs44, it necessitated a longer incubation period with bacteria to observe a similar super-infection effect in HBECs. To investigate the function of CD47 in super-infection, we reduced CD47 expression by transfecting airway epithelial cells with lentiviral shRNA targeting the CD47 gene. This resulted in a decrease in CD47 transcript levels to 56.1 ± 4.4% in HNECs (Supplementary Fig. 5a) and 58.6 ± 3.6% in HBECs (Fig. 2a), accompanied by a corresponding reduction in CD47 protein levels, 41.7 ± 5.2% in HNECs (Supplementary Fig. 5b) and 54.2 ± 6.3% in HBECs (Fig. 2b). Subsequently, we assessed the impact of CD47 knockdown on the response to super-infection by measuring paracellular FITC-dextran permeability and TEER at 3 dpi, which revealed a substantial attenuation of these effects in both HNECs (Supplementary Fig. 5c, d) and HBECs (Fig. 2c, d) following CD47 shRNA treatment. Furthermore, we inhibited CD47 protein function by pre-incubating cells with α-hCD47 neutralizing antibodies before super-infection. This intervention resulted in significant mitigation of disruption in paracellular permeability and TEER at 3 dpi in HNECs (Supplementary Fig. 5e, f) and HBECs (Fig. 2c, d), as well as a reduction in the cytopathogenic effects of super-infection in HNECs at 7 dpi (Supplementary Fig. 5g) and HBECs at 5 dpi (Fig. 2e). In a super-infection model with S. pneumoniae, the treatment of CD47 shRNA and α-hCD47 antibodies exhibited comparable protective effects on paracellular permeability and TEER in HBECs (Supplementary Fig. 6a, b). These results suggest that viral infection-induced epithelial CD47 may be exploited by pathogenic bacteria to induce super-infection by disrupting cellular junction integrity.
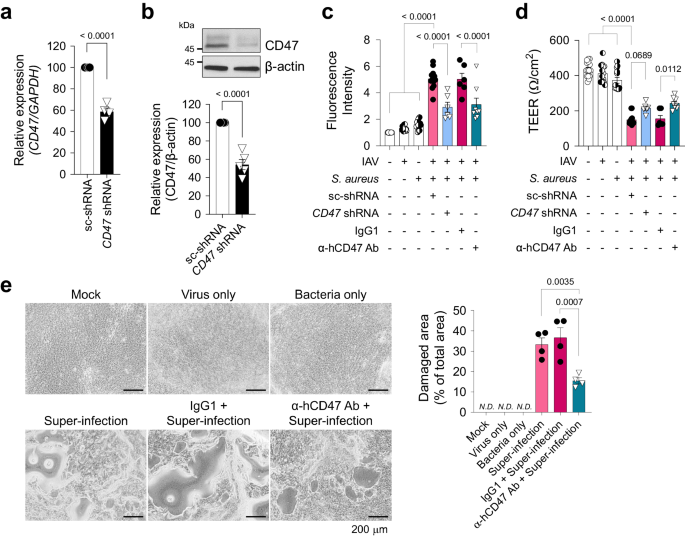
For CD47 knock-down, HBECs were transfected with scrambled shRNA (sc-shRNA) or shRNA targeting CD47 (CD47 shRNA) using a lentiviral delivery system. For CD47 neutralization, HBECs were treated with either IgG1 (MOPC-21) or α-hCD47 (B6H12.2) antibodies. a Gene expression of CD47 was analyzed using qRT-PCR (normalized by GAPDH mRNA) (sc-shRNA, n = 4; CD47 shRNA, n = 4). b Protein expression of CD47 was analyzed using immunoblotting (sc-shRNA, n = 5; CD47 shRNA, n = 5). c Paracellular FITC-dextran permeability was measured in Mock (n = 16), Virus only (IAV, n = 16), Bacteria only (S. aureus, n = 16), sc-shRNA + Super-infection (n = 12), CD47 shRNA + Super-infection (n = 6), IgG1 + Super-infection (n = 6), and α-hCD47 antibodies (Ab) + Super-infection (n = 8). d Trans-epithelial resistance was measured in Mock (n = 15), IAV (n = 14), S. aureus (n = 13), sc-shRNA + Super-infection (n = 13), CD47 shRNA + Super-infection (n = 6), IgG1 + Super-infection (n = 6), and α-hCD47 Ab + Super-infection (n = 8). e Microscopic images of HBECs at 5 dpi. The percentage of the damaged area is presented as bar graphs (Mock, n = 3; Virus only, n = 3; Bacteria only, n = 3; Super-infection, n = 4; IgG1 + Super-infection, n = 4; α-hCD47 Ab + Super-infection, n = 4). Data are presented as mean values ± SEM. Significance was determined by unpaired two-tailed Student's t test or one-way ANOVA with Tukey's multiple comparisons test. N.D. not determined. Source data are provided as a Source Data file.
Direct interactions between epithelial CD47 and the bacterial FnBP in viral-bacterial co-infected cells
With the exception of live S. aureus, no other factors, such as S. aureus-cultured media supernatant containing secreted proteins or extracellular vesicles (S), UV-killed S. aureus with intact structural proteins (U), or heat-killed S. aureus with denatured proteins (H), disrupted paracellular FITC-dextran permeability of HBECs infected with influenza virus (Supplementary Fig. 7a, Fig. 3a, b). A whole-mount image of viral-infected HBECs briefly exposed to S. aureus (for 3 h, as longer exposure led to cell layer destruction) revealed the co-localization of bacteria with CD47 on the cell surface where ZO-1 disruption occurred (Fig. 3c). This observation led to our hypothesis that live S. aureus directly interacts with host CD47 to cause super-infection. To validate this hypothesis, we initially conducted a bacterial adhesion assay to quantify the number of bacteria adhering to HBECs after a 3-hour co-incubation. This analysis revealed a significant increase in the adherence of S. aureus to the cells following viral infection in HBECs (Fig. 3d). Additionally, the virus-induced bacterial adherence was inhibited when the cells were pre-incubated with α-hCD47 antibodies (Fig. 3d), suggesting that this cell-to-bacteria interaction may be dependent on CD47.
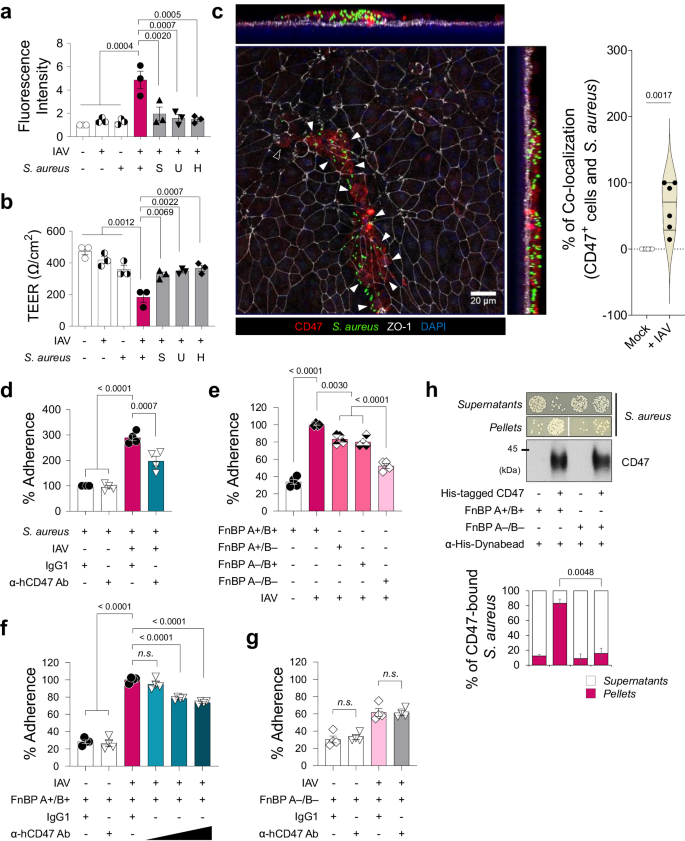
a, b Influenza virus-infected HBECs (IAV) infected with live S. aureus or S. aureus-derived samples (S, supernatant of S. aureus-cultured media; U, UV-killed S. aureus; H, heat-killed S. aureus). Paracellular FITC-dextran permeability (a) and trans-epithelial electrical resistance (b) of 7 groups: i) Mock (n = 3), ii) Virus only (n = 3), iii) Bacteria only (n = 3), iv) Super-infection (n = 3), v) Virus with S (n = 3), vi) Virus with U (n = 3), and vii) Virus with H (n = 3). c Whole mount image of CD47 (red) and S. aureus (green) in the influenza virus-infected HBECs at 1 dpi. An open arrow head indicates CD47+ cell without S. aureus and closed arrow heads indicate CD47+ cells with S. aureus. Co-localization of CD47+ cells and S. aureus are presented as violin plots (Mock, n = 6; + IAV, n = 6). d–g Bacterial adhesion assay. Colonization of S. aureus was assessed in HBECs inoculated with the virus (MOI 1) for 2 h before treatment with IgG1 control antibodies or α-hCD47 neutralizing antibodies (2 h), followed by S. aureus (MOI 3) infection for 3 h (IgG1 + S. aureus, n = 4; α-hCD47 Ab + S. aureus, n = 4; IgG1 + Super-infection, n = 4; α-hCD47 Ab+ Super-infection, n = 4) (d). Adherence of S. aureus WT (FnBP A+/B+, n = 4) and three mutant strains (FnBP A+/B–, n = 4; FnBP A–/B+, n = 4; FnBP A–/B–, n = 4) was assessed in HBECs inoculated with the virus (MOI 1) for 2 h, followed by S. aureus (MOI 3) infection for 3 h (e). Colonization of S. aureus WT (FnBP A+/B+) (f) and double deletion mutant (FnBP A–/B–) (g) was assessed in HBECs inoculated with the virus (MOI 1) for 2 h before treatment with IgG1 control antibodies (n = 4) or α-hCD47 neutralizing antibodies (1, 5, and 10 μg/mL for FnBP A+/B+, and 10 μg/mL for FnBP A–/B–, n = 4 each) for 2 h, followed by S. aureus (MOI 3) infection for 3 h. h Pull-down assay using His-tagged hCD47 recombinant protein. Bacterial plating [FnBP A+/B+ and FnBP A–/B– (n = 3 each in the absence or in the presence of His-tagged hCD47)] and immunoblot analysis were performed using supernatants and pellets after separation with α-His-Dynabeads™/DynaMag™−2 system. The graphs present the percentage of colony numbers grown in the culture of the supernatants or the pellets. Data are presented as mean values ± SEM. Significance was determined by one-way ANOVA with Tukey's multiple comparisons test or unpaired two-tailed Student's t test. n.s. not significant. Source data are provided as a Source Data file.
In our preliminary study, designed to elucidate the mechanistic basis how bacteria causing pneumonia following a secondary bacterial infection bind to CD47, we examined whether other pneumonia-causing bacteria bind to CD47. This was assessed by testing the adhesion of S. aureus, S. pneumoniae and Pseudomonas aeruginosa on A549 cells, with the commensal bacterium, Staphylococcus epidermidis serving as a negative control. The results showed that adherence of pneumonia-causing gram-positive bacteria S. aureus and S. pneumoniae to cellular CD47 was significantly inhibited following pre-incubation of the cells with α-hCD47 antibodies. However, adherence of pneumonia-causing gram-negative bacterium P. aeruginosa, was not affected by α-hCD47 antibodies. Moreover, the gram-positive commensal bacterium, S. epidermidis, did not bind to the cells (Supplementary Fig. 7b). Since only S. aureus and S. pneumoniae showed CD47-specific binding, we hypothesized that these two pathogens might share CD47-interacting components. Literature indicated that during infection, S. aureus and S. pneumoniae utilize structurally homologous adhesion molecules, such as Fn-binding protein (FnBP), to interact with integrin α5β1 by using Fn as a bridge. Specifically, FnBP A or B of S. aureus and pneumococcal adherence and virulence factor A (PavA) of S. pneumoniae are involved in these interactions4,45,46.
To test whether FnBPs are required for S. aureus binding to CD47, we employed S. aureus laboratory strain 8325-4 (FnBP A+/B+) and three mutant strains (FnBP A+/B–, A–/B+, and A–/B–)47. In bacterial adhesion assay, we observed that the single deletion of FnBP A or B reduced S. aureus adherence by 23.7 ± 1.7% or 24.7 ± 2.9%, respectively, while the double deletion of FnBP A and B synergically decreased adherence by 35.9 ± 2.4% (Fig. 3e). In contrast to the FnBP A+/B+ strain, where adherence to the viral infection-induced CD47 was inhibited by α-hCD47 antibodies in a concentration-dependent manner (Fig. 3f), the double deletion mutant strain (FnBP A–/B–) showed less adherence to viral infection-induced CD47, and this adherence was not affected by CD47 blocking (Fig. 3g). These findings indicated that FnBP A+/B+ binds to the surface of HBECs through CD47-specific (26.4 ± 1.9%) or non-specific interaction (73.6 ± 1.0%), whereas FnBP A–/B– only by non-specific interaction (61.8 ± 2.6%), suggesting a partial but direct interaction between CD47 and FnBP. To further support our hypothesis, we established an in vitro pull-down assay in which S. aureus was incubated with recombinant His-tagged hCD47 proteins and then pulled down using α-His-Dynabeads. Our results indicated a substantial increase in the precipitation of S. aureus in the pellets and a decreased presence in the supernatants when recombinant hCD47 was present, compared to the condition in which hCD47 was absent (Supplementary Fig. 7c), confirming that S. aureus directly binds to CD47. Lastly, to validate the direct interaction between CD47 and FnBP, we performed a pull-down assay using FnBP A+/B+ and FnBP A–/B–. Our results demonstrated that significantly less FnBP A–/B– (15.8 ± 6.9%) was precipitated in the pellets, even in the presence of recombinant hCD47, in contrast to FnBP A+/B+ (83.2 ± 5.7%) (Fig. 3h). By conducting in vitro pull-down assay using five mutant strains of S. aureus, in which cell wall-anchored proteins were deleted; fnbB::Tn (ΔfnbB), sasG::Tn (ΔsasG), isdB::Tn (ΔisdB), sdrE::Tn (ΔsdrE), and clfA::Tn (ΔclfA). We confirmed that only FnBP mediates the interaction between S. aureus and CD47 (Supplementary Fig. 7d, e). This observation supports the conclusion that S. aureus directly binds to HBECs through a specific interaction between FnBP and CD47.
Foxj1Cre-specific disruption or neutralization of CD47 protects mice from super-infection
Considering the increased expression of CD47 in the tracheal and lung epithelium (Supplementary Fig. 8a–e) and the decreased expression of ZO-1 in the lung lysates of C57BL/6 WT mice following infection with influenza virus (Supplementary Fig. 8f), we subsequently investigated the pathophysiological role of viral infection-induced CD47. As CD47 is ubiquitously expressed, understanding the contributions of specific CD47-expressing cell types during super-infection is crucial for gaining detailed mechanistic insights. Given that epithelial CD47 was exclusively induced in ciliated cells during viral infection (Supplementary Fig. 2d, Fig. 1e), we generated mice with selective CD47 loss in ciliated cells by creating CD47 floxed mice (CD47f/f), bred to constitutively express Cre under the control of the Foxj1 promoter (Foxj1Cre)48. Cre specificity was validated by crossing with tdTomato reporter mice (Supplementary Fig. 9a), in which Foxj1tdTomato-expressing cells were co-stained with the ciliated cell-specific marker protein Ac-α-tubulin. Immunofluorescence confirmed the specific loss of CD47 in ciliated cells of CD47FoxJ1 mice (Supplementary Fig. 9b). To ensure that CD47 inhibition during viral infection does not enhance innate immunity nor induce quicker virus clearance31, we also generated mice with selective loss of CD47 in myeloid immune cells using LysMCre mice (CD47LysM). Notably, CD47FoxJ1 and CD47LysM mice did not exhibit differences in respiratory function under baseline conditions, compared with CD47f/f mice (Supplementary Fig. 9c).
To investigate the necessity of airway epithelial CD47 in the context of viral-bacterial co-infection, we established two in vivo mouse models of super-infection. As depicted in Supplementary Figs. 10a and 10k, we employed two different concentrations of virus and bacteria in both the CD47 gene deletion experiment (100 PFU of virus and 1 ×108 CFU of bacteria) and the CD47 neutralizing experiment (10 PFU of virus and 5 ×105 CFU of bacteria). Four groups of mice, including an uninfected group (Mock), a viral infection group, a bacterial infection group, and a super-infection group, were daily monitored for alterations in body weight and mortality over a 29-day period (Supplementary Fig. 10b, c, l, m). Remarkably, approximately 98% of the mice succumbed to the super-infection within 10 days, experiencing unrecoverable weight loss. To understand the underlying causes of this lethality, we assessed i) histological damage scores of lung sections, ii) total cell numbers in bronchoalveolar lavage fluids (BALF), iii) bacterial burden in the lung and the spleen, vi) concentrations of total protein, and v) levels of pro-inflammatory cytokines (TNF-α and IL-6) in BAL fluids at 24 h following bacterial infection. In all these parameters, the super-infection group exhibited significantly increased values compared to the mock, viral infection, and bacterial infection groups (Supplementary Fig. 10e–j).
Subsequently, we investigated whether the presence of airway epithelial CD47 or myeloid CD47 is essential for super-infection. We conducted experiments using 6–8-weeks-old mice weighing between 18–21 grams with specific genetic modifications: FoxJ1-Cre;floxed (Cd47Foxj1), LysM-Cre;floxed (Cd47LysM), and control floxed (Cd47f/f) mice. These mice were infected with 100 PFU of influenza virus on day 0, followed by an infection of 1 ×108 CFU of S. aureus on day 7. Body weight loss and survival rates were monitored for 29 days. Notably, CD47FoxJ1 mice exhibited a recovery in body weight loss and improved survival rates compared to CD47f/f mice (Fig. 4a, b), while CD47LysM mice did not show the same improvements (Fig. 4k, l). Moreover, signs of pneumonia were significantly alleviated in CD47FoxJ1 mice (Fig. 4d–j) but not in CD47LysM mice (Fig. 4n–t) when compared to CD47f/f mice. This improvement was evident through several measures: i) a reduced histological lung injury score (Fig. 4c), ii) decreased total cell numbers in BALF (Fig. 4d), iii) diminished bacterial adherence and invasion in the lung (Fig. 4e, f, respectively) and bacterial burden in the spleen (Fig. 4g), iv) reduced total protein levels (Fig. 4h), and v) decreased levels of TNF-α and IL-6 in BALF 24 h after bacterial infection (Fig. 4i, j, respectively). These findings underscore the protective effect of inhibiting CD47 specifically in ciliated cells rather than myeloid cells against super-infection.
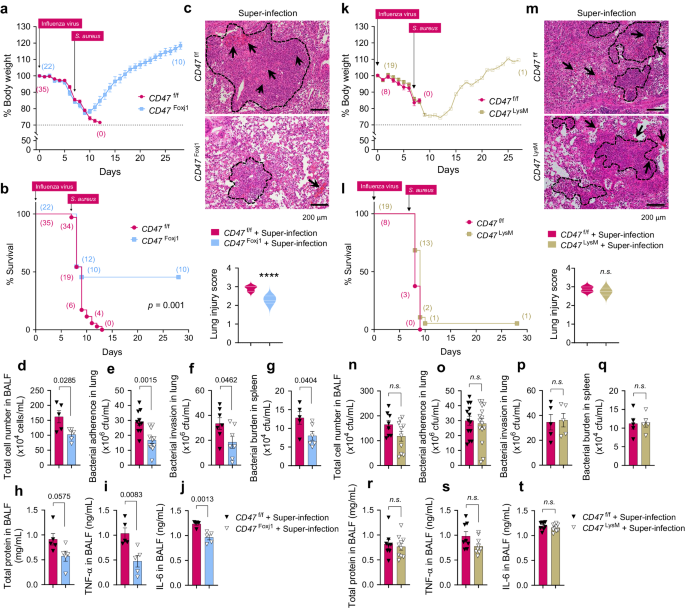
6–8-weeks-old (18–21 g of body weight) FoxJ1-Cre;floxed (CD47Foxj1), LysM-Cre;floxed (CD47LysM), and control floxed (CD47f/f) mice were infected with 100 PFU of influenza virus on day 0, and 1 ×108 CFU of S. aureus on day 7. a, b, k, l Body weight loss (a, k) and survival rates (b, l) were monitored for 29 days. The dotted line indicates the body weight exclusion cut-off. A mantel cox survival analysis was used to compare the survival rates between groups. The numbers in parenthesis represent the count of surviving mice. c, m Representative hematoxylin and eosin (H&E) staining of lung sections. The dotted lines indicate lymphocytic infiltration and arrows indicate alveolar hemorrhage. Lung injury scores are presented as violin plots in CD47Foxj1 mice (CD47f/f, n = 8; CD47Foxj1 n = 5) (c) and CD47LysM mice (CD47f/f, n = 8; CD47LysM, n = 8) (m). d–j, n–t Tissue injury parameters were measured at 24 h after bacterial infection; total cell number in BAL fluids (BALF) of CD47Foxj1 mice (CD47f/f, n = 5; CD47Foxj1, n = 5) (d) and CD47LysM mice (CD47f/f, n = 8; CD47LysM, n = 10) (n); bacterial adherence in the lung of CD47Foxj1 mice (CD47f/f, n = 10; CD47Foxj1, n = 11) (e) and CD47LysM mice (CD47f/f, n = 13; CD47LysM, n = 15) (o); bacterial invasion in the lung of CD47Foxj1 mice (CD47f/f, n = 7; CD47Foxj1, n = 7) (f) and CD47LysM mice (CD47f/f, n = 5; CD47LysM, n = 5) (p); and bacterial burden in the spleen of CD47Foxj1 mice (CD47f/f, n = 5; CD47Foxj1, n = 6) (g) and CD47LysM mice (CD47f/f, n = 5; CD47LysM, n = 5) (q); total protein concentrations in BALF of CD47Foxj1 mice (CD47f/f, n = 5; CD47Foxj1, n = 5) (h) and CD47LysM mice (CD47f/f, n = 8; CD47LysM, n = 10) (r); and inflammatory cytokines TNF-α in BALF of CD47Foxj1 mice (CD47f/f, n = 5; CD47Foxj1, n = 5) (i) and CD47LysM mice (CD47f/f, n = 8; CD47LysM, n = 10) (s), and IL-6 in BALF of CD47Foxj1 mice (CD47f/f, n = 5; CD47Foxj1, n = 5) (j) and CD47LysM mice (CD47f/f, n = 8; CD47LysM, n = 10) (t). Data are presented as mean values ± SEM. Significance was determined by unpaired two-tailed Student's t test. Source data are provided as a Source Data file.