Environmental health aspects and microbial infections of the ... - BMC Public Health
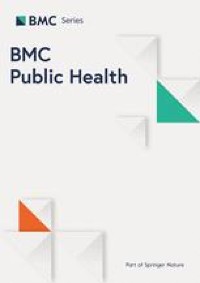
Physicochemical parameters including Cl2, TDS, pH, alkalinity, TH, Ca, and Mg showed great fluctuations in all the investigated swimming pools. Most samples violated the 1995 Egyptian Ministry of Health Decree no 418 standards. This was in accordance with a previous study done [23] in Alexandria in 2012 concluded that none of the surveyed swimming pools was met the Egyptian Standards for Swimming Pool Water no. 418/1995. Iranian research [32] recorded higher rates of pH, decreased Ca, temperature, and alkalinity all of them met Iranian standard. However free residual chlorine was lower and the mean TH was higher than the standard.
Most of the swimming pool water samples (92.3%) did not meet Egyptian bacteriological criteria. This conclusion is comparable with those of other studies in Alexandria, where 92.2–94.4 percent of water samples were bacteriologically unacceptable [3]. In Ghana, Amman, and Palestine, all water tests didn't meet bacteriological requirements [33,34,35]. Noncompliance was due to poor maintenance, operator training, disinfection, and evaluation. In other studies, lower percentages were reported in Alexandria (43.3%), Italy (34.2%), and Greece (32.9%) [23, 36, 37]. In a study conducted a year before and during the Olympic Games in Athens 2004, swimming pool noncompliance was found to be reduced from 16% in 2003 to 0% in August 2004. This study's outcome emphasizes the necessity of regular pool inspection [38].
Regarding the bacteriological parameters, the current study showed that 7.7%, 78.2%, and 100% of the surveyed samples complied with the Egyptian standard of TCC (< 100CFU/ml), TC (0 CFU /100 ml), and E. coli (0 CFU /100 ml), respectively. However, only 28.2% and 11.5% of these samples were complying with the residual chlorine (1-1.5ppm) and pH levels (7.2–7.8), as determined by the Egyptian standards, respectively. The high bacteriologically unaccepted water samples in the present study were attributed to the contamination by TCC > 100 CFU/ml, since only 7.7% of the examined swimming pool water samples complied with the Egyptian standards regarding TCC (< 100 CFU/ml). Some of the swimming pools in Alexandria showed better percentages (30.6–68%) [3].
Also, in the present study, 78.2% of water samples did not exceed the Egyptian limit for TC (0 CFU/100 ml). Lower rates were recorded in studies done in Alexandria (47%-57%) and Amman (43%) [3, 34] as well as in Iran (9.0%) and Greece (32.9%) [32, 37]. In Alexandria (2012), TC was also found in all samples and MPN/100 ml > 3.0 in 43.3% of water samples [23]. Different sample sizes, swimming pools, and disinfection techniques may explain these varying percentages.
In this study, none of the water samples examined revealed E. coli. However, other studies detected E. coli in 2.6% -8% of the samples inspected [3, 36]. In Iranian pools, the proportion was 18.2% [39]. An extremely high fecal contamination percentage was detected in Amman swimming pools, where fecal coliforms were detected in 94.7% of the samples containing TC. This shooting result was explained by a lack of pre-swimming baths, foot disinfection, and poor disinfection by untrained pool employees [34].
No difference was noted in the present study regarding the percentage of positive TCC (≥ 100CFU/ml) and TC (≥ 1CFU/100 ml) associated with different chlorine levels. This result shows that with residual chlorine above the Egyptian standard (> 1.5 ppm), TCC (≥ 100CFU/100 ml) and TC (≥ 1 CFU/100ml) represented 72.2% and 76.5%, respectively, compared to 27.8% and 23.5% with a standard chlorine level of 1-1.5 ppm. The current study revealed that TCC and TC have a positive correlation between the number of swimmers and temperature; but only TCC shows a significant correlation with pH. For years, chlorine has been the most applicable disinfectant for swimming pools. However, it must reach a certain level to be effective as a disinfectant. The accepted range of residual chlorine is 1 -1.5 ppm according to the Egyptian standard adopted by the Egyptian Ministry of Health Decree no. 418/1995. This study demonstrated that only 28.2% of the studied swimming pools' water samples complied with the Egyptian standard regarding chlorine level, whereas 71.8% had unacceptable chlorine levels. This result was similar to those previously reported in Alexandria, where 80% -88.4% of the pools had unacceptable levels of chlorine [23, 40]. However, lower percentages (56% -61.1%) of noncomplying water samples were also reported in Alexandria by Masoud in 2016 [3]. All unacceptable chlorine levels in this study were above the permissible level (> 1.5 ppm), with a mean of 2.68 ppm. This was close to that reported previously by Hamid et al. (1993) [41], who measured the chlorine level in different swimming pools in Alexandria and found that the mean residual chlorine was 2.8 mg/l. The high chlorine level recorded in this study may explain the absence of chlorine sensitive organisms, such as E. coli from all water samples. Different results were reported in other studies showing lower chlorine levels among unaccepted pools' water in Egypt and Iraq [23, 42]. Additionally, other studies have demonstrated higher and lower residual chlorine levels among noncomplying pools [34, 40]. This high variability of recorded residual chlorine levels indicates the variable amount of chlorine added to the pools (operator dependent) and lack of continuous monitoring and maintenance of pools by the authority.
However, despite high residual chlorine levels, high TCC (> 100 CFU/ml) was demonstrated in 72.2% of the studied water samples. This result may be explained by the presence of chlorine resistant bacteria (CRB). CRB are frequently described as bacteria that exhibit high resistance to chlorine disinfection or bacteria which may persist or regrow within the residual chlorine. Therefore, complete control of CRB infectivity cannot be achieved through chlorine disinfection. Another study focused on CRB pathogenicity and antibiotic resistance. Researchers found that Mycobacterium, Bacillus, Legionella, Pseudomonas and Sphingomonas are among the commonest pathogenic CRB genera, which are mainly pathogenic. However, nonpathogenic CRB were overlooked by other researchers [43, 44]. In a previous study, there was an overexpression of antibiotic resistant genes in some CRB which tolerates different chlorine levels, indicating a chlorine-associated induction of antibiotic resistance in the pathogen [43].
Mucoid Pseudomonas aeruginosa strains survive and regenerate better in chlorinated pool water than nonmucoid cultures. This was explained by overexpression of the extracellular polysaccharide layer that acts as a barrier against the disinfecting effect of chlorine [45]. In addition, Jin et al. (2020) [46] showed that chlorinated water improved plasmid transfer through genetic transformation. These transfer organelles were unaffected by chlorination, resulting in antibiotic-resistant bacteria. Environmental pollutants, CODMn, NH4+–N, and metal ions accelerated this reaction. The present study showed that 100% and 38.6% of the collected samples during the dry season, were positive for TCC and TC, respectively, whereas 82.4% and none of the samples collected in the wet season were positive for TCC and TC, respectively, with no statistically significant difference.
Globally, bathing in swimming pools is a channel for infection transmission; therefore, evaluation of parasitic infections in relation to physicochemical parameters pools' water is mandatory [47]. It had been shown that mismanaged swimming pools may result in waterborne diseases [32]. The contamination of pools with pathogenic protozoa poses a serious threat to bathers [48]. In the current study, a PI rate of 73.1% was recorded in the water of studied swimming pools. Intestinal coccidia showed the highest rate of infections (Cyclospra & Isospora 37.2%, and Cryptosporidium spp., 34.6%), which may be attributed to their abilities to survive for months at ambient temperatures in moist environments [49]. This was followed by G. lamblia, 21.8%; Blastocystis spp., 14.1%; and Microsporidia spp., 15.4%. Lower rates of infections (≤ 2.6%) were reported for E. coli, and E. histolytica. Meanwhile, Acanthameba spp., rate was 5.1%.
High rates of Cryptosporidium spp. were detected in swimming pools in Italy and the United States (40% and 55.6%, respectively) [50, 51]. In contrast, other studies done in Italy and Netherlands revealed lower rates (28.6% and 4.6%, respectively) [52, 53]. Recently, Chalmers et al. [54] detected Cryptosporidium oocysts in 12/59 (20%) of pool water samples and increased to 8/12 (66%) in pool samples collected in August when bather loads were highest. The study done by Abd El-Salam in 2012 reported a low rate of both Cryptosporidium and G. lamblia (10%) in the five swimming pools selected randomly from different districts in Alexandria [23]. Meanwhile, these two parasites were detected with rates of (16.7% and 15.0%, respectively) in the 60 water samples collected from 35 swimming pools in Beijing, China [55]. Lower rates of G. lamblia were even detected in the Netherlands and United States (5.9% and 5.6%, respectively) [51, 53]. Contrarily, two studies done in Italy in 2004 and 2006 recorded high G. lamblia infection rates (40% and 28.6%, respectively) [50, 52]. The high percentage of contamination might be attributed to high bathers' load/day, young-aged swimmers, and not having a shower before swimming, all these factors contributed to shedding of pathogens in the water [50].
Fournier et al. [56] reported Cryptosporidia spp. and Microsporidia spp. from one out of the 48 samples collected from six swimming pools in Paris, France, while giardiasis wasn't detected. Additionally, Galván et al. (2013) [57] estimated a rate of 9% of Cyclospra in the different water sources examined including recreational water.
Several studies in Iran detected higher rates of Acanthameba spp. Than the rate reported by the present study. In 2017, Mafi [58] reported 24% as a rate for Acanthameba spp. in pool water and amusement parks' pool; meanwhile Solgi et al. and Sarmadian et al. [59, 60], revealed percentages of 20% and 16.7% respectively, in swimming pools. The difference in these studies was attributed to an increase in the water temperature of hot springs.
The present work showed that dry season, heavy daily bathers' load/h (250–500 bathers/h), higher temperatures (27C), longer duration of swimming pool work (> 10 h/day), turnover of 6 hrs, abnormal pH and alkalinity are associated with higher rate of water contamination by parasites. Superficially collected samples, decreased cleaning frequency, highly circulated water samples (≥ 80 m3/min), Cl2 > 1.5 ppm, TDS ≥ 1500 ppm, and TH > 400 mg/l were proved to be significant risk factors for parasitic contamination of pools' water. Multivariate logistic regression evaluating physicochemical parameters linked with parasite water contamination revealed that the highest risk variables were pool cleaning frequency, water flow rate, Cl2 and TDS. The increased bather load, extended pool work hours, and infrequent cleaning, altogether, increase both fecal and nonfecal water contamination resulting in high rated of PI [61]. Zangiabadi et al. (2011) [61] reported that the simultaneous use of pools by bathers is associated with microbial contamination risk and subsequent diseases. In 2008, WHO reported that as the temperature increases to more than 27°C, microbial activity would increase accordingly [62]. Reduction in the turnover rate results in increasing the flow rate, which subsequently do not remove particles leading to lower filtration efficiency [63, 64]. In China, it was reported that the rate of Cryptosporidium was higher in August than in May (24.2% and 7.4%, respectively) [55]. In the done in UK, 2021, oocysts were detected in 12/59 (20%) pool water samples, 66% of them were detected in August [54]. Similar data was reported by Sheilds et al., [65] and they attributed it to a high density of bathers due to the hot weather in August in Beijing. The same was found in USA and Urmia, with higher protozoan and fungal contamination recorded during summer for the same reasons, in addition to the high temperature and humidity in this season [66, 67].
Concerning chemical parameters, this study revealed a high parasitic contamination of water in spite of the achieved desired residual chlorine level, and this could be attributed to parasitic resistance to chlorination [61, 62, 68, 69]. Hence, the desirability of physicochemical parameters could be ineffective in removing the parasites even if they were in the standard level, and this agreed with Rabi et al. [34]. A high level of TDS could reduce the chlorine efficacy and could cause dulling in pools' water clarity [70].
In conclusion, the current comprehensive study revealed that the tested water samples don't meet the Egyptian bacteriological criteria and about 73% of samples examined were contaminated with parasites in spite that desired chlorine level was achieved. The most common pathogens recorded were intestinal coccidia, G. lamblia, Microsporidia spp., and Blastocystis spp. Acanthameba spp. was also detected but at a low rate. Thus, monitoring the swimming pool's water quality and improving the disinfection system are both mandatory. Government safety programs should incorporate health education about swimming hygiene.
Comments
Post a Comment