LOX-1 acts as an N6-methyladenosine-regulated receptor for Helicobacter pylori by binding to the bacterial catalase - Nature.com
Abstract
The role of N6-methyladenosine (m6A) modification of host mRNA during bacterial infection is unclear. Here, we show that Helicobacter pylori infection upregulates host m6A methylases and increases m6A levels in gastric epithelial cells. Reducing m6A methylase activity via hemizygotic deletion of methylase-encoding gene Mettl3 in mice, or via small interfering RNAs targeting m6A methylases, enhances H. pylori colonization. We identify LOX-1 mRNA as a key m6A-regulated target during H. pylori infection. m6A modification destabilizes LOX-1 mRNA and reduces LOX-1 protein levels. LOX-1 acts as a membrane receptor for H. pylori catalase and contributes to bacterial adhesion. Pharmacological inhibition of LOX-1, or genetic ablation of Lox-1, reduces H. pylori colonization. Moreover, deletion of the bacterial catalase gene decreases adhesion of H. pylori to human gastric sections. Our results indicate that m6A modification of host LOX-1 mRNA contributes to protection against H. pylori infection by downregulating LOX-1 and thus reducing H. pylori adhesion.
Similar content being viewed by others
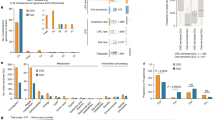
A distinct Fusobacterium nucleatum clade dominates the colorectal cancer niche
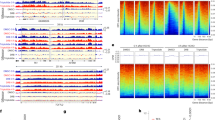
Endogenous aldehyde-induced DNA–protein crosslinks are resolved by transcription-coupled repair
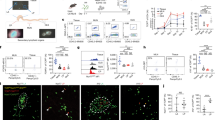
Immune microniches shape intestinal Treg function
Introduction
Helicobacter pylori is a microaerophilic Gram-negative pathogen that colonizes the stomach of over 50% of the world population, with a higher prevalence in countries with lower socio-economic status1,2. Chronic infection with this pathogen is a predominant driver for a broad range of clinical sequelae in susceptible individuals, including chronic gastritis, peptic ulcer, mucosa-associated lymphoid tissue lymphoma, and gastric adenocarcinoma3. The infection is usually acquired in childhood and persists lifelong, unless eradicated by antibiotics-based treatments4. According to the consensus of the 2015 Kyoto conference, all H. pylori-positive individuals should receive eradication therapy, unless there are competing reasons5. However, the eradication of this pathogen has been increasingly difficult owing to the globally increasing antibiotic resistance over the last few decades, with the treatment failure rates now reaching 10 to 30%6,7. Since 2017, H. pylori has been listed by the World Health Organization as a high-priority pathogen posing serious threat to the human health8. Thus, a better understanding of the fundamental mechanism underlying H. pylori infection as well as developing novel treatment strategies are urgently needed.
N6-methyladenosine (m6A) RNA methylation is the most prevalent internal messenger RNA (mRNA) modification in eukaryotes, occurring in approximately 0.1–0.4% of adenosines and predominantly located in 3′-untranslated regions (3′-UTRs), near stop codons, and in terminal exons of over 25% of human transcripts9. Owing to the rapid advancement of m6A-mapping methods, m6A modification is now recognized as a pivotal regulatory mechanism controlling gene expression10. m6A RNA methylation is a reversible and dynamic process, finely tuned by the opposing activities of m6A methyltransferases (also known as "writers", including methyltransferase-like 3 (METTL3), methyltransferase-like 14 (METTL14), and Wilms tumor 1 associated protein (WTAP)) and m6A demethylases (also known as "erasers", including fat-mass and obesity-associated protein (FTO) or alkylation repair homolog protein 5 (ALKBH5))11. To execute the biological functions of m6A modification, m6A-modified mRNAs have to be "read" by YT521-B homology (YTH) domain-containing proteins, including YTHDF1-3, YTHDC1 and YTHDC2 (also known as "readers"), which regulate RNA stability, alterative splicing, nuclear export, and translational efficiency12. Prevailing evidence indicates that m6A modification is key to the regulation of diverse physiological processes, including cell differentiation, inflammation, autophagy, and viral infection13,14,15,16. However, the role of m6A modification in H. pylori infection has not been investigated.
In the current study, we discovered that m6A modification was increased in H. pylori-infected gastric epithelium, due to upregulation of m6A "writers". Such elevation in m6A level then restricted the colonization of H. pylori. Moreover, by an integrative analysis of m6A-sequencing (m6A-seq) and RNA-sequencing (RNA-seq), we identified Lectin-Like Oxidized Low-Density Lipoprotein Receptor-1 (LOX-1) as the direct downstream target of m6A modification.
Results
H. pylori infection up-regulated cellular m6A "writers" and m6A level
To delineate the interactions between H. pylori infection and cellular m6A modifications, we first determined the expression levels of principal m6A "writers" in H. pylori-positive and -negative human gastric specimens (Supplementary Table 1 for patients' demographic data). Immunohistochemical (IHC) staining revealed that the expression levels of METTL3, METTL14 and WTAP were all significantly upregulated in H. pylori-positive samples when compared to the H. pylori-negative specimens. In line with the upregulation of m6A "writers", cellular m6A levels were also increased in H. pylori-positive samples (Fig. 1a). Given m6A modification is a reversible process, we determined gastric m6A levels in H. pylori-infected patients before and after H. pylori eradication with antibiotics. The m6A dot-blot assay showed that the total m6A levels were decreased in 10 out of 12 pairs of patients' specimens after receiving antibiotic treatment (Supplementary Fig. 1; Supplementary Table 2 for patients' demographic data). In the next step, we orally inoculated C57BL/6 mice with H. pylori Sydney strain 1 (SS1) for 3 months and the mouse stomachs were collected to examine the expression levels of major m6A "writers" and "erasers". Similar to the human gastric specimens, Mettl3, Mettl14 and Wtap but not Fto or Alkbh5 were markedly increased in H. pylori-infected mouse samples (Fig. 1b), accompanied by elevated cellular m6A levels as measured by the m6A dot blot assay (n = 13 per group; 5 samples per group shown in Fig. 1c and 8 additional samples per group shown in Supplementary Fig. 2). For in vitro study, two H. pylori strains (TN2GF4 and ATCC 43504) were used to co-culture with the human gastric epithelial cells HFE14517,18, and the expression levels of major m6A "writers" and "erasers" were determined by Western blots. The results showed that METTL3, METTL14 and WTAP were remarkedly upregulated, while FTO and ALKBH5 were slightly increased in HFE145 cells during H. pylori infection in a time-dependent manner (Fig. 1d), and the cellular m6A level was also significantly increased after co-culturing with H. pylori (Fig. 1e).
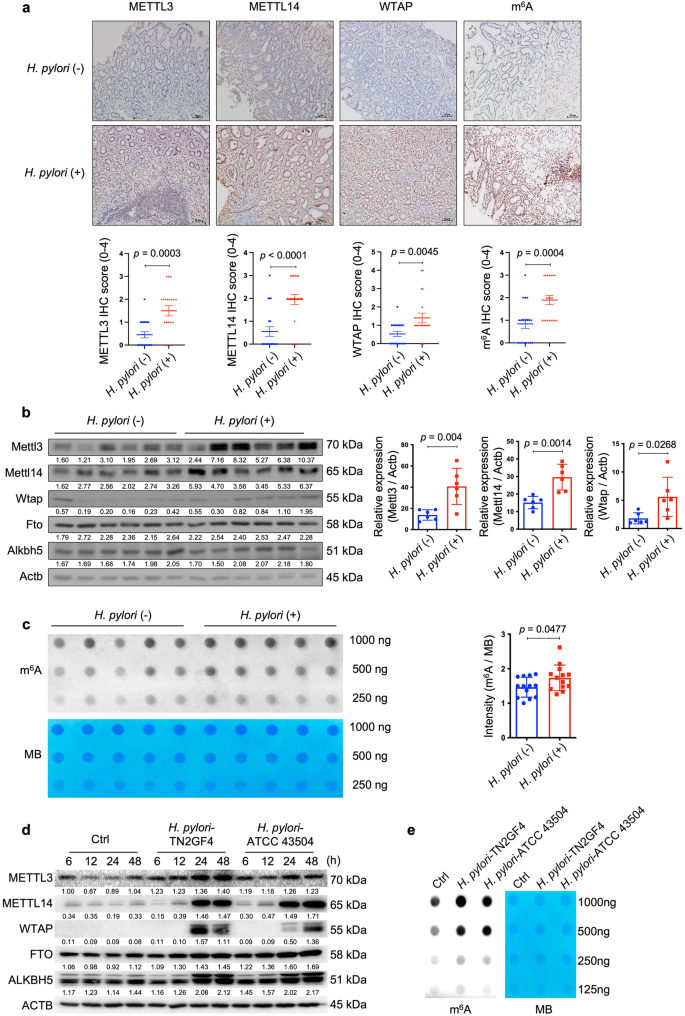
a Representative images of immunohistochemical (IHC) staining for METTL3, METTL14, WTAP and cellular m6A levels in H. pylori-positive (n = 20 samples) and -negative (n = 20 samples) human gastric specimens. Quantitative analysis was shown as means ± SD. Scale bar, 20 μm. b Total proteins were extracted from mouse stomach tissues of the control group (n = 6 animals) and H. pylori-infected group (n = 6 animals), and the expression levels of Mettl3, Mettl14, Wtap, Fto and Alknh5 were examined by Western blots. Actb was used as a loading control. The gray scales of each sample were measured by Image J and the quantitative analysis of target/Actb was conducted. Quantitative analysis was shown as means ± SD. c The m6A level of poly(A)+ RNAs in mouse stomach specimens with (n = 5 animals) or without (n = 5 animals) H. pylori colonization was evaluated by m6A dot blot assay. The RNA from each sample was loaded equally by a 2-fold serial dilution. MB staining was used as a loading control. The intensity of dots was measured by Image J and quantitative analysis was shown as m6A/MB (n = 13 per group in total; in addition to the 5 samples per group shown in this figure, 8 extra samples per group were shown in Supplementary Fig. 2). Quantitative analysis was shown as means ± SD. d Human gastric epithelial cells HFE145 were co-cultured with two H. pylori strains (TN2GF4, ATCC 43504; MOI = 100). The cells were harvested at different time points (6, 12, 24, 48 h) to measure the protein levels of m6A "writers" (METTL3, METTL14, WTAP) and "erasers" (FTO, ALKBH5) by Western blots. ACTB was used as a loading control. The blots shown are representative of three independent experiments with similar results. e HFE145 cells were co-cultured with two H. pylori strains (TN2GF4, ATCC 43504; MOI = 100) for 24 h. Poly(A)+ RNA from total RNA was isolated form H. pylori-infected HFE145 cells. The Poly(A)+ RNA was loaded onto the membrane with a 2-fold serial dilution. MB staining was used as a loading control. The blots shown are representative of three independent experiments with similar results. Statistical analysis of the data (a–c) was performed using unpaired two-tailed Student's t test and the corresponding p-values are included in the figure panels. Source data are provided as a Source Data file.
Ablation of m6A writers enhanced whereas overexpression of m6A writers reduced H. pylori infection
Next, we speculated whether the change of cellular m6A level was involved in the defense against H. pylori infection. To answer this question, we first employed Mettl3+/- mice to determine whether the hemizygous deletion of the principal m6A "writer" could affect H. pylori colonization in vivo. Wild-type and Mettl3+/− mice were orally inoculated with H. pylori strain SS1 for 3 months, and the bacterial colonization in the mouse stomachs was examined by colony formation assay and immunofluorescence. As shown in Fig. 2a, b, the colonization of H. pylori in Mettl3+/− mouse stomachs was remarkedly increased when compared to wild-type mice, suggesting that the downregulation of cellular m6A level could promote H. pylori infection. We and other investigators previously reported that H. pylori invaded into the gastric epithelial cells could serve as a source for chronic infection19,20,21,22,23. The effect of m6A ablation on intracellular colonization of H. pylori was then explored in vitro. Two individual siRNAs targeting major m6A "writers" (METTL3, METTL14 and WTAP) were respectively transfected into HFE145 cells to ablate cellular m6A levels (Fig. 2c). The transfected cells were further co-cultured with H. pylori for 6 h, followed by incubation with gentamycin for 2 h to kill the extracellular bacteria. The intracellular colonization of H. pylori was then examined by H. pylori-specific 16S ribosomal DNA (16S rDNA) qPCR, immunofluorescence, and colony formation assay. These experiments collectively showed that knockdown of major m6A "writers" significantly increased the intracellular abundance of H. pylori (Fig. 2d–f). Furthermore, we overexpressed these major m6A "writers" (METTL3, METTL14, WTAP) in HFE145 cells to increase the cellular m6A levels (Fig. 2g). Consistently, the intracellular colonization of H. pylori was decreased in the m6A "writers"-overexpressing cells as shown by all three quantitative assays, namely, 16S rDNA qPCR, immunofluorescence, and colony formation assay (Fig. 2h–j).
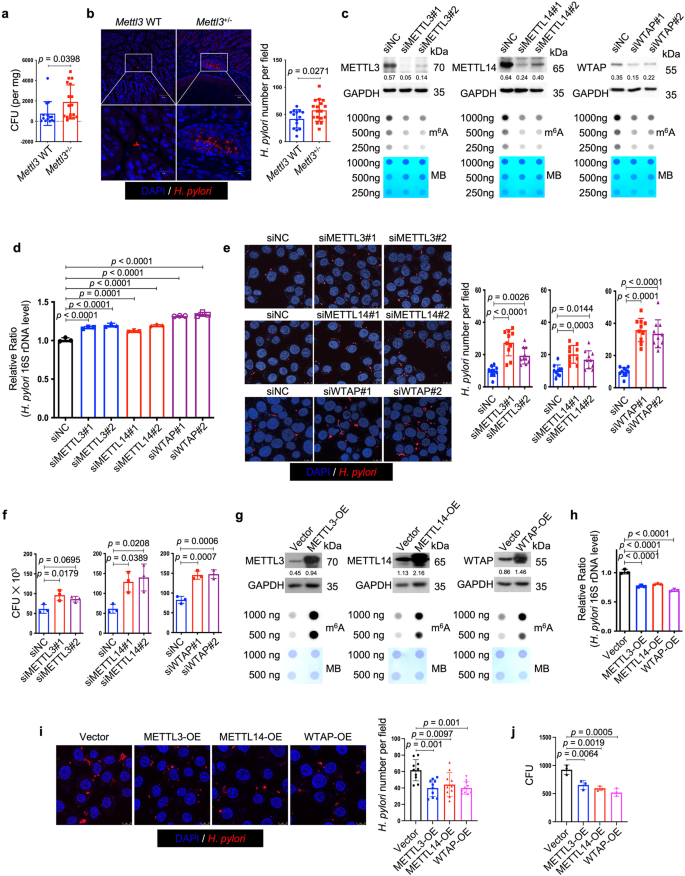
a, b Wild type (n = 14 animals) and Mettl3+/− mice (n = 17 animals) were infected with H. pylori strain SS1 for 3 months. a Mouse stomach tissues were harvested and weighted, and then homogenized in sterile PBS. The samples were further diluted and spread on H. pylori-selective blood agar plates. After growing for 5 days, the colony numbers were count for quantitative analysis. b Parafilm-embedded sections of mouse stomach were stained to visualize H. pylori (Red) and the nuclei (Blue). Ten visual fields of each sample were randomly selected to count H. pylori number. Scale bar, 50 μm. c, g Two individual siRNAs or overexpression plasmids were transfected into HFE145 cells to knock down or overexpress METTL3, METTL14 and WTAP, respectively. The knockdown or overexpression efficiency was examined by Western blots. GAPDH served as a loading control. The m6A level of poly(A)+ RNA was examined by Dot blot. Methylene blue (MB) staining was used as a loading control. d–f, h–j HFE145 cells transfected with siRNAs or overexpression plasmids were infected with H. pylori (MOI = 100) for 24 h. d, h Intracellular H. pylori 16S ribosomal DNA levels were measured by real-time PCR. Human GAPDH was used as an internal control (n = 3 replicates for each group). e, i Cells were stained to visualize invaded H. pylori (Red) and the nuclei (Blue). Ten visual fields of each group were randomly selected to count H. pylori number. f, j Cells were permeabilized with 1% saponin for 15 min. The diluted samples were then spread on H. pylori-selective blood agar plates and incubated for 5 days to count the colony number (n = 3 replicates for each group). All the quantitative data were shown as means ± SD. Statistical analysis of the data was performed using unpaired two-tailed Student's t test (a, b) or one-way ANOVA (d–f, h, i, j) followed by Tukey's multiple comparison tests with adjustments, and the corresponding p-values are included in the figure panels. The statistical significance of the data (d–f, h, i, j) was calculated from one of three independent experiments with similar results. Source data are provided as a Source Data file.
LOX-1 mRNA was identified as an m6A-modified transcript in H. pylori infection
To identify the m6A-modified target(s) which was involved in the modulation of H. pylori infection, m6A-seq and RNA-seq analysis were performed in HFE145 cells with or without H. pylori infection. Results showed that m6A modification of the transcript of Lectin-Like Oxidized Low-Density Lipoprotein Receptor 1 (LOX-1), also known as Oxidized Low Density Lipoprotein Receptor 1 (OLR1), was highly upregulated while the mRNA level of LOX-1 was significantly downregulated upon H. pylori infection (Supplementary Figs. 3 and 4). We next examined the data of m6A-seq and RNA-seq in H. pylori-infected HFE145 cells with or without WTAP knockdown. Integrative analysis of m6A-seq and RNA-seq showed that the m6A level of LOX-1 was remarkedly decreased accompanied by an increase in mRNA level upon knockdown of WTAP in H. pylori-infected HFE145 cells (Fig. 3a). The distribution of m6A peaks on LOX-1 mRNA was further visualized by the Integrative Genomics Viewer (IGV) tool, which revealed the marked enrichment of m6A in the 3ʹ-UTR of LOX-1 mRNA upon H. pylori infection whereas WTAP knockdown markedly reduced the m6A levels and increased the mRNA level of LOX-1 (Fig. 3b). As reported, LOX-1 serves as a scavenger receptor that mediates oxidized low‐density lipoprotein internalization through receptor-mediated endocytosis24. Besides, LOX-1 could act as a receptor that promotes the adhesion of Escherichia coli and Mycobacterium tuberculosis to macrophages and mediates the bacterial internalization25,26. Therefore, we speculated that LOX-1 might be an m6A-regulated target that modulated H. pylori attachment and invasion into gastric epithelial cells. The m6A modification and transcriptional alteration of LOX-1 were next validated by m6A-qRT-PCR and qRT-PCR, respectively, and the related protein alteration of LOX1 was further confirmed by Western blots (Fig. 3c–e). In addition, the mRNA and protein levels of LOX-1 were upregulated upon knockdown of METTL3, METTL14 or WTAP, confirming that LOX-1 expression was negatively regulated by m6A modification (Fig. 3f, g). Moreover, RNA decay assay showed that knockdown of METTL3, METTL14 or WTAP increased the mRNA stability of LOX-1 (Fig. 3h). To further confirm LOX-1 was regulated by m6A modification on its 3ʹ-UTR, dual luciferase assay was performed using LOX-1 3ʹ-UTR with or without mutation of the m6A sites. As expected, knockdown of m6A "writers" upregulated the luciferase activity, whereas mutation of m6A sites on the LOX-1 3ʹ-UTR abolished such effects (Fig. 3i).
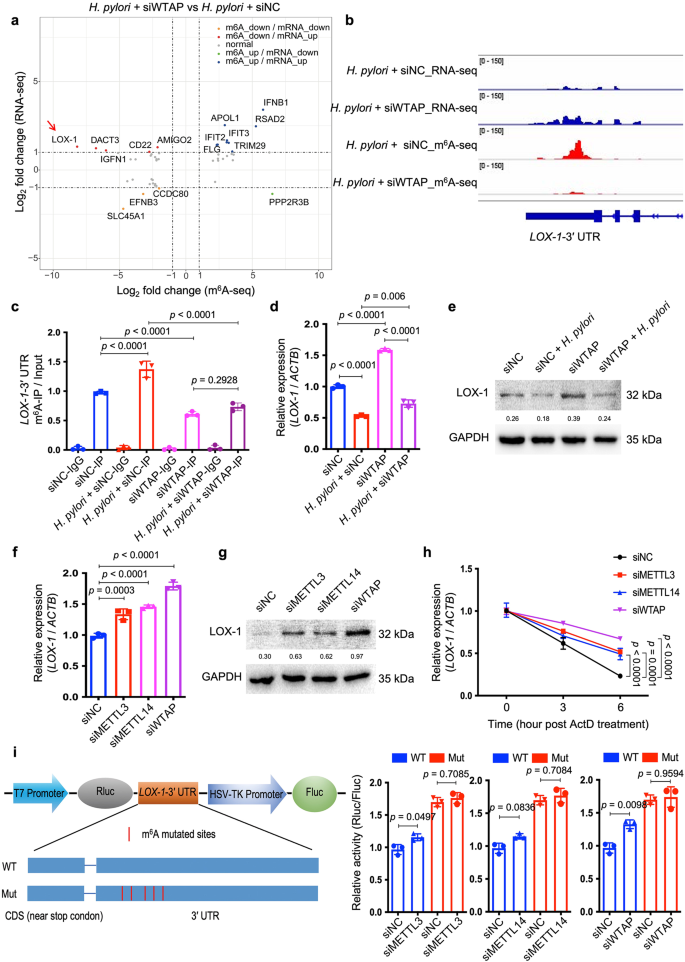
a Integrated analysis of m6A-seq and RNA-seq from H. pylori-infected HFE145 cells transfected with negative control or WTAP siRNAs was shown. The fold change of m6A-seq was normalized to the corresponding transcript expression level. Genes with Log2 fold change > 1 were highlighted. b The m6A peaks located at 3′UTR of LOX-1 in m6A-seq and the corresponding peaks in RNA-seq were visualized by IGV tool. c–e HFE145 cells transfected with negative control or WTAP siRNAs were infected with or without H. pylori for 24 h. c MeRIP-quantitative PCR was performed to validate m6A enrichment on LOX-1-3′UTR. The m6A enrichment of each group was calculated by m6A-IP/input (n = 3 replicates for each group). d LOX-1 mRNA levels of each group were measured by real-time PCR. Human ACTB was used as an internal control (n = 3 replicates for each group). e LOX-1 protein levels of each group were examined by Western blots. Human GAPDH was used as a loading control. f–h HFE145 cells transfected with negative control or siRNAs targeting METTL3/METTL14/WTAP were infected with H. pylori for 24 h. f LOX-1 mRNA levels of each group were measured by real-time PCR. Human ACTB was used as an internal control (n = 3 replicates for each group). g LOX-1 protein levels of each group were examined by Western blots. Human GAPDH was used as a loading control. h RNA decay rates of LOX-1 of each group were measured after treating with Actinomycin D (normalize to 0 h, n = 3 replicates for each group). i Two luciferase plasmids were constructed by inserting corresponding CDS into pmiR-RB-ReportTM vector. The wild type plasmid contained the full-length 3′UTR of LOX-1 and partial CDS near the stop codon, whereas five m6A-consensus motifs identified from m6A-seq were mutated with A-to-C conversion in the mutated plasmid. The relative luciferase activity was measured and calculated by normalizing Renilla to Firefly activity (n = 3 replicates for each group). All the quantitative data were shown as means ± SD. Statistical analysis of the data was performed using one-way ANOVA (c, d, f, h, i) followed by Tukey's multiple comparison tests with adjustments and the corresponding p-values are included in the figure panels. The statistical significance of the data (c, d, f, h, i) was calculated from one of three independent experiments with similar results. Source data are provided as a Source Data file.
LOX-1 served as a cell surface protein to promote the adhesion and invasion of H. pylori into gastric epithelial cells by binding to the bacteria catalase
To delineate the function of LOX-1 in H. pylori infection, we first used two individual siRNAs to knock down LOX-1 in HFE145 cells (Fig. 4a). Knockdown of LOX-1 significantly reduced the intracellular colonization of H. pylori (Fig. 4b–d), whereas overexpressing LOX-1 showed an opposite effect (Fig. 4e–h). Moreover, silencing LOX-1 abolished the effect of WTAP knockdown-mediated increase in bacterial load in HFE145 cells, indicating that LOX-1 was an important downstream effector in m6A-regulated H. pylori infection (Fig. 4i). To further confirm the functional role of LOX-1 in vivo. Wild-type and Lox-1−/− mice were infected with H. pylori for 1 month, and the bacterial colonization in the mouse stomachs was examined by colony formation assay and immunofluorescence. As shown in Fig. 4j–k, H. pylori colonization was significantly decreased in Lox-1−/− mice. We also observed the colocalization between Lox-1 and H. pylori in wild-type mice while these colocalized signals disappeared in Lox-1−/− mice, suggesting that Lox-1 might serve as a cell surface receptor for H. pylori adhesion and colonization. Moreover, hematoxylin and eosin (H&E) staining of mouse gastric specimens was performed, confirming that the inflammatory cell infiltration was reduced in Lox-1−/− mice upon H. pylori infection (Supplementary Fig. 5). By expressing GFP-LOX-1 and staining H. pylori in HFE145 cells, we also observed the colocalized signals on cell surface in vitro, which further confirmed the interactions between LOX-1 and H. pylori (Fig. 5a). Moreover, bacterial adhesion assay was performed, and the results showed that LOX-1 knockdown decreased the adhesion levels of H. pylori to HFE145 cells, whereas overexpression of LOX-1 showed an opposite effect, suggesting that LOX-1 could act as a cell membrane protein to support H. pylori attachment on gastric epithelial cells (Fig. 5b, c). Next, we sought to identify which outer membrane protein(s) of H. pylori could interact with LOX-1. To achieve this, we first co-incubated the H. pylori-extracted proteins with HFE145 cell lysates. Then, specific LOX-1 antibody was used to pull down LOX-1 as well as its interacted proteins from the mixture, and the enriched proteins were further separated on SDS-PAGE followed by silver staining (Fig. 5d). A specific protein band above the IgG heavy chain was identified as the catalase of H. pylori by mass spectrometry analysis (Fig. 5d). Furthermore, we performed reciprocal coimmunoprecipitation to validate the direct interaction between LOX-1 and H. pylori catalase. As expected, we confirmed the direct binding between LOX-1 and catalase (Fig. 5e).
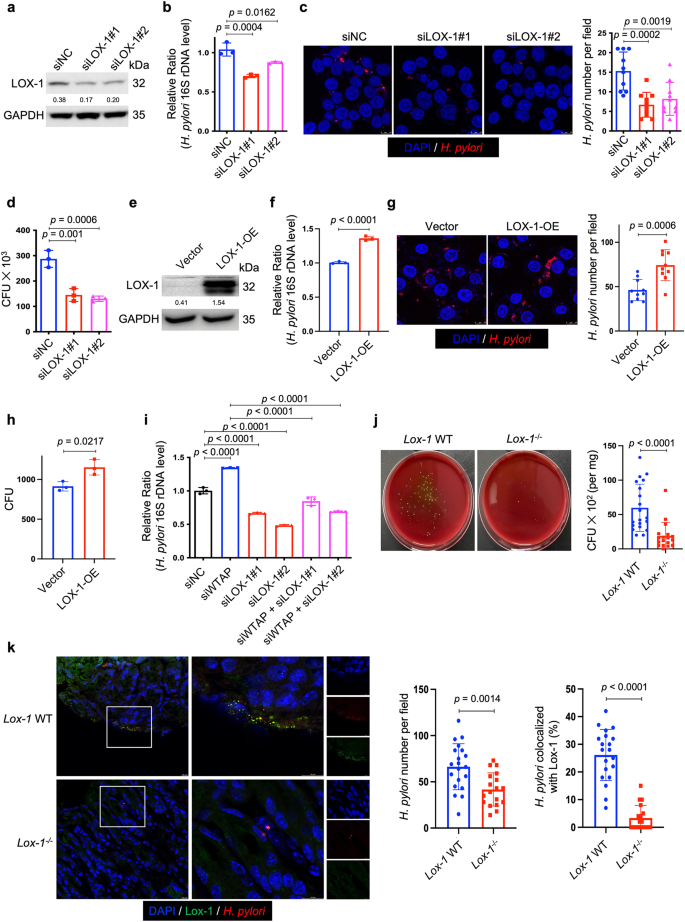
a, e Two individual siRNAs or overexpression plasmids were transfected into HFE145 cells to knock down or overexpress LOX-1. The knockdown or overexpression efficiency was validated by Western blots. GAPDH was served as a loading control. b–d, f–h HFE145 cells transfected with siRNAs or overexpression vector of LOX-1 were infected with H. pylori (MOI = 100) for 24 h. b, f Intracellular H. pylori 16S ribosome DNA levels were measured by real-time PCR. Human GAPDH was used as an internal control (n = 3 replicates for each group). c, g Cells were stained to visualize invaded H. pylori (Red) and nuclei (Blue). Ten visual fields of each group were randomly selected to count H. pylori number. d, h Cells were permeabilized with 1% saponin for 15 min. The diluted samples were then spread on H. pylori-selective blood agar plates and incubated for 5 days to count the colony number (n = 3 replicates for each group). i HFE145 cells transfected with WTAP siRNAs and/or LOX-1 siRNAs were infected with H. pylori (MOI = 100) for 24 h. Intracellular H. pylori 16S ribosomal DNA levels were measured by real-time PCR. Human GAPDH was used as an internal control (n = 3 replicates for each group). j, k Wild type (n = 20 animals) and Lox-1−/− mice (n = 18 animals) were infected with H. pylori strain SS1 for 1 month. j Mouse stomach tissues were harvested and weighted, and then homogenized in sterile PBS. The samples were further diluted and spread on H. pylori-selective blood agar plates. After growing for 5 days, the colony numbers were count for quantitative analysis. k Parafilm-embedded sections of mouse stomach were stained to visualize Lox-1 (green), H. pylori (Red) and the nuclei (Blue). Ten visual fields of each sample were randomly selected to count H. pylori number and measure the colocalized signals of H. pylori and Lox-1. Scale bar, 10 μm. All the quantitative data were shown as means ± SD. Statistical analysis of the data was performed using unpaired two-tailed Student's t test (f, g, h, j, k) or one-way ANOVA (b–d, i) followed by Tukey's multiple comparison tests with adjustments, and the corresponding p-values are included in the figure panels. The statistical significance of the data (b–d, f, g–i) was calculated from one of three independent experiments with similar results. Source data are provided as a Source Data file.
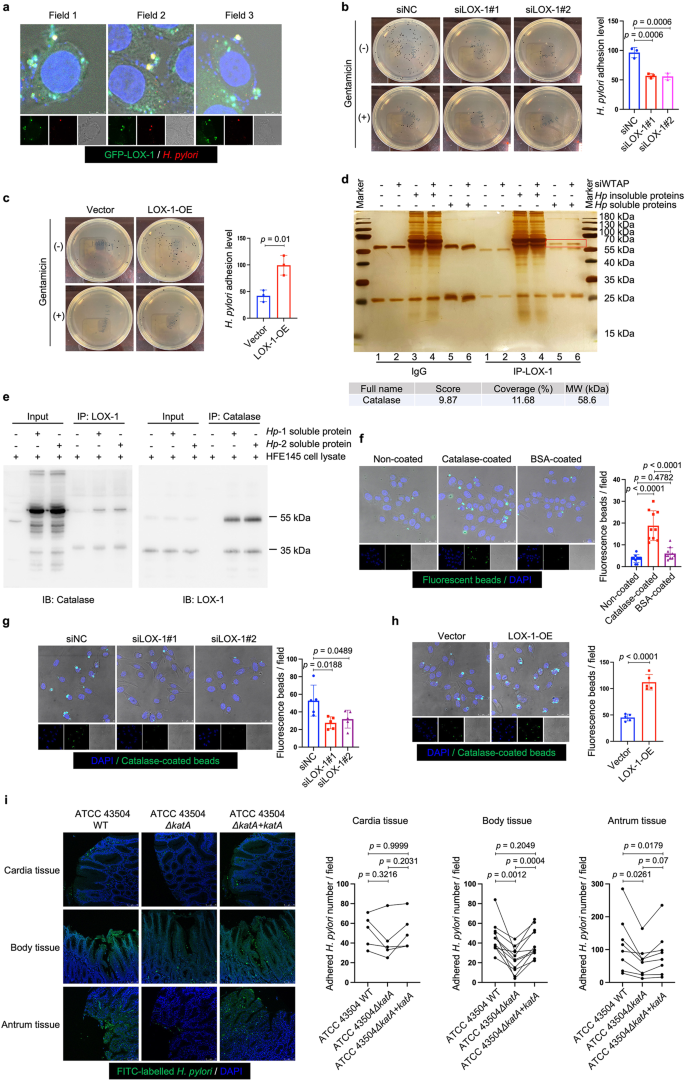
a HFE145 cells expressing GFP-LOX-1 (green) protein were infected with H. pylori (MOI = 100) for 6 h, and cells were further stained to visualize H. pylori (red). The representative visual fields shown are representative of three independent experiments with similar results. Scale bar, 5 μm. b, c HFE145 cells transfected with siRNAs or overexpression plasmid of LOX-1 were infected with H. pylori (MOI = 100) for 6 h, followed by treating with or without 200 μg/ml gentamycin for 2 h. Cells were permeabilized with 1% saponin for 15 min. The diluted samples were then spread on H. pylori-selective blood agar plates and incubated for 5 days to count the colony number. H. pylori adhesion levels were calculated by subtracting the CFU of group with gentamycin treatment from the CFU of the group without (n = 3 replicates for each group). d The lysates of HFE145 cells transfected with negative control or WTAP siRNAs were incubated with soluble or insoluble proteins of H. pylori. A specific antibody was used to pull down LOX-1 and its interacting proteins from the incubated protein mixture. The pulled down proteins were separated in SDS-PAGE followed by silver staining. The red square indicates a protein band that was only observed in the LOX-1-pulled down groups incubated with H. pylori soluble proteins. Mass spectrum analysis revealed the specific protein band as H. pylori catalase. The gel shown is representative of three independent experiments with similar results. e HFE145 cell lysate was incubated with the soluble proteins of two H. pylori strains (TN2GF4 and ATCC 43504), and LOX-1 or catalase antibody was used to perform reciprocal co-immunoprecipitation assay. The predicted molecular size of catalase is 60 kDa, and the molecular size of LOX-1 is 50 kDa (mature form) and 32 kDa (precursor). The blots shown are representative of three independent experiments with similar results. f HFE145 cells were incubated with non-coated, catalase-coated, or BSA-coated fluorescent beads (green) for 6 h (MOI = 100), and cells were stained to visualize nuclei (blue). Ten visual fields of each group were randomly selected to count the attached and internalized fluorescent beads. g, h HFE145 cells transfected with siRNAs or overexpression vector of LOX-1 were incubated with catalase-coated fluorescent beads (MOI 100) for 6 h and were stained to visualize nuclei (blue). Ten visual fields of each group were randomly selected to count the attached and internalized fluorescent beads. Scale bar, 25 μm. i FITC-labeled H. pylori strains (wild-type, ΔkatA and ΔkatA + katA strains) were respectively incubated with human normal stomach tissue array (72 tissue cores from 24 cases (i.e., triplicate sections for each case), US Biomax, BN01011B). Each line represents the binding of the three H. pylori strains in an individual patient as measured by calculating the mean number of adhered H. pylori on triplicate sections, respectively. Quantitative analysis of the bacteria binding to different gastric areas (cardia tissues from 5 cases, gastric body tissues from 11 cases, and gastric antrum tissues from 8 cases) were performed, respectively. Scale bar, 75 μm. All the quantitative data were shown as means ± SD. Statistical analysis of the data was performed using unpaired two-tailed Student's t test (c, h) or one-way ANOVA (b, f, g, i) followed by Tukey's multiple comparison tests with adjustments, and the corresponding p-values are included in the figure panels. The statistical significance of the data (b–d, f–h) was calculated from one of three independent experiments with similar results. Source data are provided as a Source Data file.
It has been reported that catalase was located on the bacterial surface during autolysis of H. pylori27. To confirm the involvement of catalase in LOX-1-mediated H. pylori adhesion, we constructed a recombinant His-tagged catalase for generating catalase-coated fluorescent beads (Supplementary Fig. 6). To simulate bacterial infection, the coated beads were incubated with HFE145 cells in a multiplicity of infection (MOI) of 1:100. The confocal data showed that catalase coating significantly promoted the adhesion and internalization of fluorescent beads in gastric epithelial cells (Fig. 5f). Moreover, knockdown of LOX-1 reduced the adhesion and internalization of catalase-coated beads, whereas overexpression of LOX-1 produced an opposite effect (Fig. 5g, h). To further confirm the bacterial catalase was indeed involved in the adhesion of H. pylori to human gastric tissues, we next constructed an isogenic catalase-negative mutant with ATCC 43504 (ΔkatA) using an allelic exchange method to inactivate the catalase-coding gene katA. Genetic complementation of katA in ΔkatA mutant (ΔkatA + katA) was also performed (Supplementary Figs. 7 and 8). To further confirm if katA knockout could only affect catalase expression but not other proteins of H. pylori, silver staining was performed to analyze the whole-cell bacterial proteins. A protein band slightly above the 55-kDa marker (the molecular weight of catalase is 58 kDa) disappeared in both soluble and insoluble protein fractions of ΔkatA strain but no obvious changes of other protein bands could be observed (Supplementary Fig. 9a). Moreover, qRT-PCR was performed to detect the mRNA expression of two major H. pylori adhesin-encoding genes (i.e., babA and sabA) in wild-type, ΔkatA and ΔkatA + katA strains. The results showed KatA knockout or its genetic supplementation did not affect babA or sabA expression (Supplementary Fig. 9b). Next, by incubating fluorescein isothiocyanate (FITC)-labeled wild-type ATCC 43504 or its ΔkatA or ΔkatA + katA strains with sections of human normal gastric tissue array (72 section cores from 24 cases (i.e., triplicate sections from each case); US Biomax, BN01011B), we found that the adhesion levels of catalase-negative mutant strain were markedly decreased in gastric body (n = 11, range and mean of inhibition: 11.4–92.0% and 48.8%) and antrum tissues (n = 8, range and mean of inhibition: 7.4–60.7% and 44.2%), and the adhesion was restored after genetic complementation of katA gene (gastric body: range and mean of restoration: 40.9–144.4% and 80.9%; gastric antrum: range and mean of restoration: 8.3–142.9% and 49.3%; with 100% restoration corresponds to the complete restoration the adhesion level back to that of the wild-type strain). However, no significant changes were observed in cardia tissues (n = 5, range and mean of inhibition: −9.9–41.1% and 18.8%; range and mean of restoration: −28.6–328.6% and 86.3%; Fig. 5i, Supplementary Fig. 10). Considering pre-heating of the tissue array (to avoid tissue detachment) at 60 °C before the staining process might denature tissue proteins, including the putative receptor(s) for bacterial adhesion, we also compared the adhesion level of the three H. pylori strains (i.e., wild-type, ΔkatA and ΔkatA + katA strains) in human gastric sections without or with pre-heating. The results showed that pre-heating at 60 °C slightly reduced H. pylori binding to the human gastric tissues but did not alter the inhibitory effect of katA knockout or the restoring effect of katA genetic complementation (Supplementary Fig. 11).
Blockage of LOX-1 by a small molecule inhibitor suppressed H. pylori infection in vitro and in vivo
A newly identified small-molecule inhibitor of LOX-1, referred to as BI-0115, was reported to selectively target LOX-1 and potently block cellular uptake of oxLDL. Combining biophysical and crystal-structure analyses, it was found that BI-0115 binding could inhibit LOX-1 by stabilizing an inactive receptor tetramer state28. Therefore, we next employed BI-0115 to determine if pharmacological blockage of LOX-1 could suppress H. pylori infection. Pre-treating HFE145 cells with 2.5 μM BI-0115 effectively reduced the number of invaded H. pylori, while this effect was not detected when using BI-1580, a control chemical analog of BI-0115 with much less drug potency (https://www.opnme.com/molecules/lox-1-bi-0115, Fig. 6a–c). Besides, BI-0115 but not BI-1580 significantly inhibited the adhesion of H. pylori to HFE145 cells (Fig. 6d). Furthermore, SS1-infected C57BL/6 mice were treated with 10 mg/kg BI-0115 or vehicle on alternate days for 2 weeks followed by colony formation assay and immunofluorescence (Fig. 6e). The results showed that BI-0115 significantly suppressed colonization of H. pylori in the mouse stomachs (Fig. 6f, g). Besides, histopathological assessment revealed the attenuation of inflammatory cell infiltration (Fig. 6h). Meanwhile, BI-0115 administration reduced the expression of the pro-inflammatory interleukin-6 (IL-6, Fig. 6i), suggesting that H. pylori-induced inflammation was attenuated in BI-0115-treated mouse stomachs. Thus, our in vitro and in vivo findings suggest that targeting LOX-1 may help reduce H. pylori colonization in the stomach.