FacZ is a GpsB-interacting protein that prevents aberrant division-site placement in Staphylococcus aureus - Nature.com
Abstract
Staphylococcus aureus is a Gram-positive pathogen responsible for antibiotic-resistant infections. To identify vulnerabilities in cell envelope biogenesis that may overcome resistance, we enriched for S. aureus transposon mutants with defects in cell surface integrity or cell division by sorting for cells that stain with propidium iodide or have increased light-scattering properties, respectively. Transposon sequencing of the sorted populations identified more than 20 previously uncharacterized factors impacting these processes. Cells inactivated for one of these proteins, factor preventing extra Z-rings (FacZ, SAOUHSC_01855), showed aberrant membrane invaginations and multiple FtsZ cytokinetic rings. These phenotypes were suppressed in mutants lacking the conserved cell-division protein GpsB, which forms an interaction hub bridging envelope biogenesis factors with the cytokinetic ring in S. aureus. FacZ was found to interact directly with GpsB in vitro and in vivo. We therefore propose that FacZ is an envelope biogenesis factor that antagonizes GpsB function to prevent aberrant division events in S. aureus.
Similar content being viewed by others
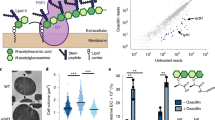
Staphylococcus aureus cell growth and division are regulated by an amidase that trims peptides from uncrosslinked peptidoglycan
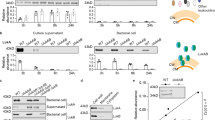
The cell envelope of Staphylococcus aureus selectively controls the sorting of virulence factors
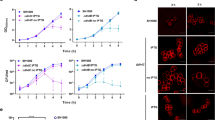
The Staphylococcus aureus cell division protein, DivIC, interacts with the cell wall and controls its biosynthesis
Main
The cell envelope of the opportunistic pathogen Staphylococcus aureus is vital for resisting turgor pressure and interfacing with the host. Like most Firmicutes, its surface is composed of a cytoplasmic membrane surrounded by a thick peptidoglycan (PG) cell wall, with anionic polymers called lipoteichoic acids and wall teichoic acids decorating the respective layers1. Because the mechanical integrity conferred by the cell envelope is necessary for survival2, the biosynthetic pathways that build it have been effective targets for many of our most successful antibiotic classes. However, many strains of S. aureus have evolved resistance to cell-wall-targeting antibiotics3. A deeper understanding of the mechanisms that promote envelope biogenesis is therefore needed to uncover new ways to target this essential structure for drug development.
In rod-shaped bacteria, envelope assembly is performed in two phases: elongation and division. Depending on the organism, elongation proceeds via the insertion of a new cell wall at dispersed sites throughout the cell cylinder or at the cell poles4. This phase is followed by cell division, which in Gram-positive bacteria involves the assembly of a multilayered cell wall septum that initially bisects the mother cell. The septum is built by the divisome, a multiprotein PG synthesis machine organized by treadmilling polymers of the tubulin-like FtsZ protein that condense into a dynamic structure called the Z-ring to establish the division site4. After the septum is built, enzymes with cell wall cleaving activity, called PG hydrolases or autolysins, split the septum to promote daughter cell separation and complete the division process5,6.
Spherical S. aureus cells do not have as distinct an elongation phase as their rod-shaped cousins7. Instead, envelope biogenesis is principally focused at the division site throughout the cell cycle8. In many rod-shaped bacteria, a combination of the Min system and nucleoid occlusion defines the division site by guiding Z-ring formation to midcell9,10,11. Although S. aureus also uses a nucleoid occlusion protein12,13, much less is known about how it regulates division-site placement to localize envelope assembly. As a sphere, S. aureus must restrict division to a single midcell plane among the theoretically infinite number of such planes available. An additional complexity in S. aureus not shared by rod-shaped cells is that its division site rotates through different perpendicular planes in successive cell cycles14. Geometric and/or structural epigenetic cues have been suggested to aid in coordinating division site rotation, but the underlying mechanism is unclear15,16. Thus, much remains to be learned about how this seemingly simple, spherical bacterium controls the biogenesis of its envelope.
To uncover new factors involved in envelope assembly in S. aureus, we performed two related comprehensive screens for mutants with altered cell morphology and/or increased envelope permeability. Hits included many genes known to be critical for proper envelope assembly and morphogenesis, indicating that the screens worked as intended. The screens also implicated a number of genes of unknown function in envelope assembly, providing a rich dataset for uncovering new insights into the process. We characterized one of these hits, facZ, and found that it encodes a regulator of cell division placement targeting the conserved cell division protein GpsB.
Results
Screens for S. aureus envelope biogenesis mutants
A high-density transposon mutant library of S. aureus (strain RN4220) was constructed and subjected to two enrichment regimens using a cell sorter (Fig. 1). The envelope defective (END) enrichment sorted for mutants with altered membrane permeability based on increased staining with propidium iodide (PI), a membrane-impermeable fluorescent DNA dye (Fig. 1a). Although PI staining is typically associated with lethal loss of membrane integrity17, we reasoned that we could enrich for mutants experiencing an increased frequency of these defects based on the association of dead, PI-positive cells with viable siblings in unseparated cells. In the related cell separation or division (CSD) enrichment, mutants defective for cell separation or cell division were sorted based on increased light scattering of larger cells or cell clusters18,19 (Fig. 1b). To set the sorting cut-offs (gates) for each enrichment, we used a Δatl mutant defective in the major cell separation autolysin5,20 as a control (Fig. 1c). As expected, the larger size and shape complexity of unseparated cell clusters of the mutant gave rise to a population of cells causing increased light scattering (ScatterHI) compared with a wild-type control. In addition, we observed the appearance of a population of PI-positive (PIHI) cells in cultures of the Δatl mutant that was largely absent in wild-type cultures (Fig. 1c). The sorting parameters for the END and CSD enrichments using the transposon library were defined to capture ScatterHI and PIHI cells with similar properties to those of the Δatl mutant, which we anticipated would enrich for mutants with a broad range of envelope biogenesis and cell division defects in addition to those impaired for cell separation (Fig. 1d).
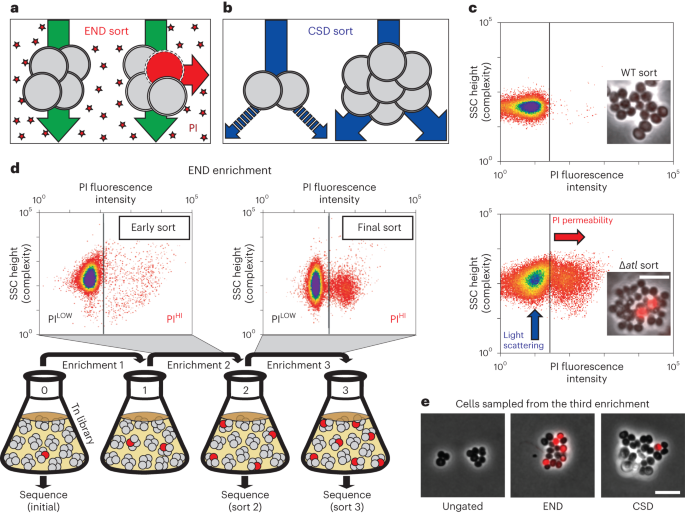
a,b, Schematics showing the logic of the END (a) and CSD (b) enrichments. See text for details. c, FACS profiles monitoring light scattering (SSC height) and PI fluorescence for wild type (WT) and Δatl strains. Inset micrographs show phase contrast images with an overlay of PI staining in red (scale bar = 4 µm; the scale bar applies to all images in c). d, Schematic detailing the END enrichment workflow. An analogous CSD enrichment was performed in parallel (not shown). See text for details. Enrichment for PIHI mutants was examined by FACS profiling at each stage (top). e, The final cell populations (strain aTB015 [RN4220 ∆attB(f11)::Orf5 pTM378]) from the END and CSD sorts, as well as an ungated control, were imaged on 2% agar pads (scale bar = 4 μm; the scale bar applies to all images in e). PI staining (red) was overlaid on the phase contrast image.
Before sorting, the transposon library was grown to mid-exponential phase. We then collected cells passing the ScatterHI or PIHI gates (106 cells for each) as well as an equivalent number of cells sorted without a gate for use as a control. The resulting sorted populations were recovered, amplified in growth medium and sorted again using the same parameters. Three sequential enrichments were performed for each population, maintaining cells in exponential phase throughout the procedure (Fig. 1d). Imaging of cells from the populations recovered following the third sort showed that the protocol was effective in enriching for cells with the desired phenotypes (Fig. 1e).
To monitor changes in the representation of transposon mutants in the library during enrichment, transposon sequencing (Tn-seq) was performed on the original unsorted library and the mutant populations following the second and third sorts for ScatterHI, PIHI or the ungated control sort. In the initial transposon library, the median gene had 17 unique insertions, and 2,468 of the 2,629 annotated genes (93.9%) had at least one insertion (Supplementary Table 1). To determine which mutants were being enriched by the sorts, the number of insertion reads at each locus from the END or CSD enrichments was compared with those in the ungated control at the same time point (Supplementary Table 2). By the second sort, we observed a subset of genes showing greater than fourfold enrichment in insertions relative to the ungated control, and this enrichment became even more pronounced by the third sort (Extended Data Fig. 1a,b). Furthermore, for each enrichment, the increased representation of insertions in a given gene following the second sort was correlated to its enrichment following the third sort (Extended Data Fig. 1c,d). Thus, each enrichment was selective, and multiple rounds of sorting yielded stronger phenotypic enrichment.
The enrichments of END and CSD mutants were moderately correlated, with many of the 50 most-enriched mutant loci for each sorting scheme overlapping (Extended Data Fig. 1e and Supplementary Table 3). To identify mutants showing the greatest combined enrichment across both sorts, we analysed the enrichment data using a two-dimensional principal component analysis (2D-PCA; Extended Data Fig. 1f). A total of 74 candidate 'hits' were enriched at least eightfold compared with unsorted controls along the first principal component, PC1, which serves as a proxy for combined enrichment in both screens (Supplementary Table 4). Among the hits were genes with known roles in envelope biogenesis, cell separation and cell division such as sepF21, atl5,20 and sagB22,23,24, with transposon insertions in these genes showing clear enrichment following both the END and CSD sorts (Fig. 2a, Extended Data Fig. 2a and Supplementary Table 5). Genes of unknown function were also well represented among the list of hits, including facZ (SAOUHSC_01855), which showed an enrichment in transposon insertions following both sorting methods, and showed aberrant cell morphology and an increased frequency of PIHI cells in cultures (Fig. 2, Extended Data Fig. 2a and Supplementary Table 5). Mutants in genes of unknown function, SAOUHSC_01975 and SAOUHSC_02383, were also identified as hits, and were shown to have defects in cell division and/or envelope permeability (Extended Data Fig. 2). Among these hits, we found facZ (SAOUHSC_01855) to be particularly interesting because of the unusual nature of the membrane and cell wall depositions observed in cells lacking FacZ (Fig. 2b). We therefore focused on investigating its function.
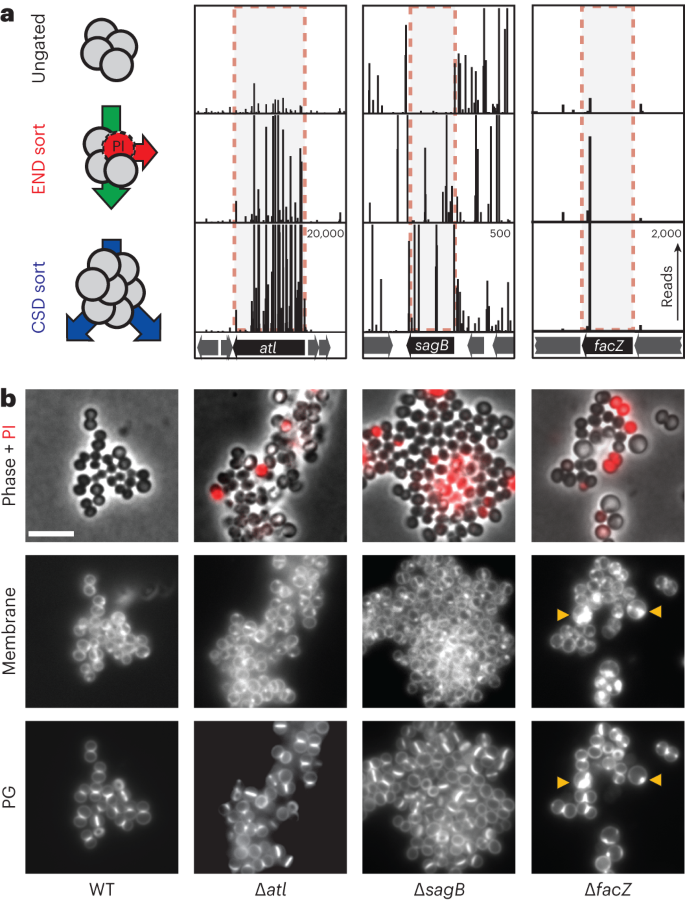
a, Tn-seq profiles from three genomic loci showing enrichment of transposon insertions at the completion of the END and CSD sorting protocols relative to the control sort. Each vertical line represents a mapped insertion site, and the height of the line is the number of reads mapping to that site, which reflects the representation of the insertion mutant in the population. Profiles for each locus are scaled separately with the maximum number of reads indicated in the top right corner of the bottom profile. b, Representative images of WT and mutant cells (∆atl [aTP103], ∆sagB [aTB287] and ∆facZ [aTB251]) pulse labelled with sBADA to visualize PG synthesis (bottom row), stained with N,N,N-trimethyl-4-(6-phenyl-1,3,5-hexatrien-1-yl)-benzenaminium, 4-methylbenzenesulfonate (TMA–DPH) to label the cell membrane ('Membrane', middle row) and treated with PI to assess envelope permeability ('Phase + PI', top row). Yellow arrowheads highlight membrane and PG synthesis defects. The fluorescence intensity for each channel was scaled identically for all strains to facilitate direct comparison between images (scale bar = 4 µm; the scale bar applies to all images in b).
facZ mutants have pleiotropic envelope defects
To further characterize the envelope defects of cells lacking FacZ, a ΔfacZ mutant was generated in the HG003 strain background and pulse labelled with the fluorescent d-amino acid (FDAA) (R)-2-Amino-3-(3-(5,5-difluoro-7,9-dimethyl-2-sulfo-5H-4λ4,5λ4-dipyrrolo[1,2-c:2',1'-f][1,3,2]diazaborinin-3-yl)propanamido)propanoic acid (sBADA) to label sites of nascent cell wall synthesis25, followed by treatment with Nile red and 2-[4-(Aminoiminomethyl)phenyl]-1H-Indole-6-carboximidamide hydrochloride (DAPI) to visualize the membrane and nucleoid, respectively. The mutant cells were more heterogeneous in size than wild-type cells and showed strikingly aberrant membrane and PG accumulations that often colocalized (Fig. 3a–c and Extended Data Fig. 3a). These accumulations excluded the nucleoid, indicating that they projected into the cytoplasmic space (Fig. 3d). Transmission electron microscopy (TEM) also revealed the aberrant accumulation of envelope material in ΔfacZ mutant cells (Fig. 3a and Extended Data Fig. 3g), and "super-resolution" three-dimensional structured illumination microscopy (3D-SIM) of labelled cells confirmed the colocalization of the membrane and PG stains, and that these projections are probably invaginations that are continuous with the peripheral cell envelope (Extended Data Fig. 3b and Supplementary Video). Cells lacking FacZ also showed aberrant localization of FtsZ–GFP. Many cells had multiple FtsZ structures oriented at oblique angles relative to one another, indicative of problems with division site placement (Extended Data Fig. 3c,d). Notably, the essential divisome PG synthesis proteins FtsW and Pbp1 localized to the aberrant envelope invaginations of ∆facZ mutants, indicating that the invaginations probably arise from misplaced divisome activity (Extended Data Fig. 3e). Expression of a chromosomally integrated copy of facZ under control of the anhydrotetracycline (aTc) inducible tet promoter (Ptet) complemented the morphological and growth defects of ΔfacZ cells, showing that the defects were caused by the lack of FacZ production and not effects of the deletion allele on the expression of nearby genes (Extended Data Fig. 3f; also see complementation assays in Extended Data Figs. 4g and 5c). We conclude from these data that FacZ is critical for normal envelope biogenesis, and that its absence results in the formation of spurious invaginations of the envelope that represent aberrant, misplaced division attempts.
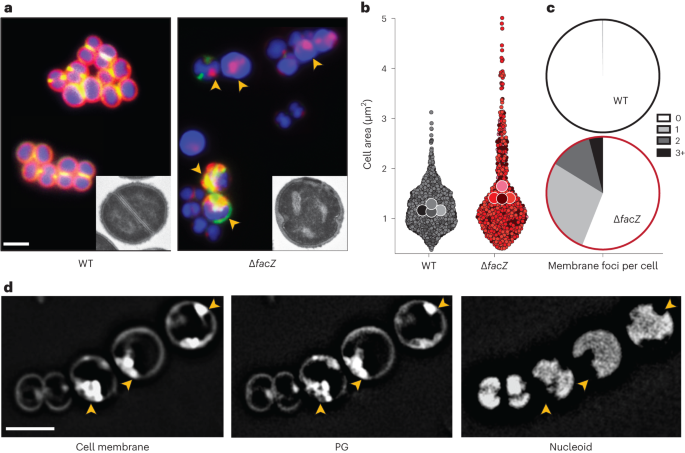
a, Cells from WT and ∆facZ strains were pulse labelled with sBADA to visualize PG synthesis (green), washed three times with PBS to arrest growth and remove unincorporated sBADA, and then labelled for 5 min with the membrane stain Nile red (red). Cells were imaged on 2% agar pads containing DAPI to visualize the nucleoid (blue). Yellow arrowheads highlight cells with aberrant membranes and PG synthesis. Insets show cells from the same strains imaged by TEM. The bright spots in the ∆facZ mutant are consistent with the cell envelope inclusions, and probably represent cell envelope projections into the cell interior (Supplementary Fig. 3b). b, Violin plots showing the cell area of the indicated strains harbouring a cytoplasmic fluorescent protein. The results from four biological replicates were pooled, each shaded differently; small circles are individual measurements, and large circles are medians from each replicate (WT = 1,760 cells, ∆facZ = 701 cells; Supplementary Fig. 3). c, Quantification of aberrant membrane foci in TMA–DPH-labelled cells (n > 200 cells; Supplementary Fig. 3a). d, 3D-SIM microscopy of ∆facZ cells stained identically to those in a. Yellow arrowheads highlight the position of aberrant cell wall and membrane accumulations that exclude the nucleoid. Scale bars = 2 µm. The scale bars apply to all images in a and d.
Source data
Periseptal localization of FacZ
FacZ is an 18 kDa protein predicted to possess an N-terminal transmembrane (TM) helix and a C-terminal cytoplasmic domain composed of a membrane-proximal coiled-coil region followed by an intrinsically disordered region with a net positive charge (Extended Data Fig. 4a,b). AlphaFold Multimer predicts that FacZ forms homo-oligomeric assemblies with three to four protomers with a well-packed interface. In such a configuration, the complex is predicted to be shaped like an anchor, extending ~15 nm into the cytoplasm with disordered C-terminal 'fingers' that extend from a helical rod (Extended Data Fig. 4c).
To determine the subcellular localization of FacZ in S. aureus, we constructed a facZ–mCherry fusion and expressed it from an ectopic locus under control of the Ptet promoter in cells that were also pulse labelled with the FDAA sBADA to monitor PG synthesis. Importantly, the fusion protein complemented the growth and morphological defects of ΔfacZ cells at the same minimal concentration of inducer (50 ng µl−1 aTc) as the analogous untagged allele (Extended Data Fig. 4d–g). All localization experiments were performed using this level of induction for the facZ–mCherry fusion. Consistent with its predicted TM helix, FacZ–mCherry showed a peripheral membrane localization, outlining all cells in the population (Fig. 4a and Extended Data Fig. 4d,e). In addition to the peripheral signal, FacZ–mCherry appeared to be enriched at sites of septum formation but confined to the periphery of the septum (the 'periseptum') even at late stages in division (Fig. 4a and Extended Data Fig. 4d,e). We quantified this enrichment by measuring two different fluorescence intensity profiles in populations of labelled cells: one through the cell body perpendicular to the septum (orthogonal profile) and the other around the cell periphery (peripheral profile) (Fig. 4c–f). In cells in the early stages of division identified by their incomplete PG septa, FacZ–mCherry showed enrichment at the edges of the orthogonal profile, and at the periseptum in the peripheral profile, where PG labelling was also most intense (Fig. 4c,d). FacZ periseptal enrichment was slightly but significantly greater (1.18-fold periseptal:peripheral signal, P < 0.001) than a non-specific membrane label (Extended Data Fig. 4f). However, due to the diffraction-limited resolution of our imaging method, the precise localization pattern underlying the periseptal FacZ–mCherry signal remains uncertain (Extended Data Fig. 4e). Regardless, the protein remained strikingly fixed at the periseptum in cells at later stages of division, as the FacZ–mCherry signal in the inner septum remained at or near baseline even in cells in which the septum appeared complete (Fig. 4c). This pattern is in stark contrast to the expected midcell enrichment of the PG and membrane stains in the orthogonal profiles of late-stage septating cells (Fig. 4e). No change was observed in the peripheral profile, as the structure of the periseptum is not expected to change until the final stage of division when cells separate26 (Fig. 4d,f). We therefore conclude that FacZ is present and modestly enriched at the periseptum, but unlike typical membrane proteins, it is excluded from the inner septal membrane.
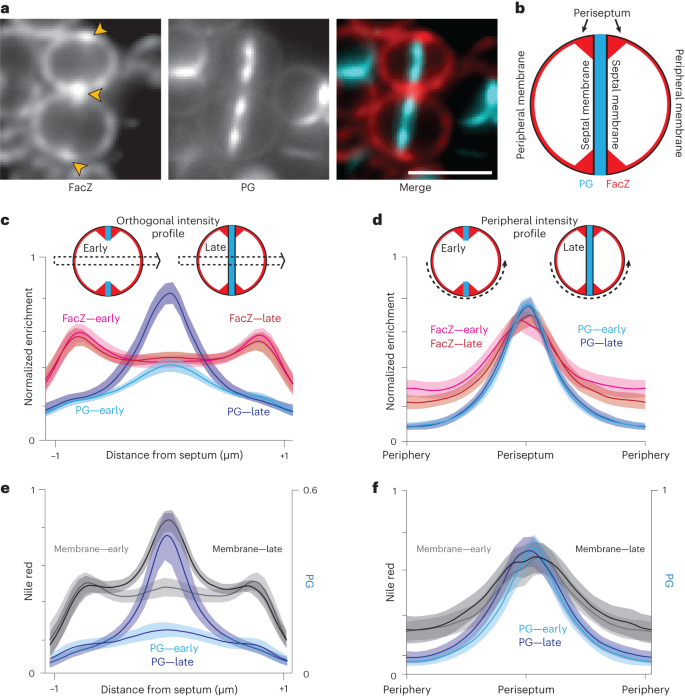
a, Representative fluorescence images of WT cells expressing FacZ–mCherry stained with HADA to label PG (scale bar, 2 μm; scale bar applies to all images in a). The protein fusion (left) and PG label (centre) and a pseudo-coloured merged image are shown (FacZ–mCherry in red, HADA in blue) (Extended Data Fig. 5e–g). b, Schematic depicting the membrane localization of FacZ in a dividing S. aureus cell. c, Graphs of mean fluorescence intensity of HADA (light and dark blue) and FacZ–mCherry (light and dark red) collected along lines perpendicular to the septum of cells labelled as in a; shaded areas represent 95% confidence intervals. Central peaks show intensity at the septum, and peripheral peaks show intensity at the cell periphery. Cells were grouped into early division (cells with two HADA foci representing an unresolved septum) or late-stage division (cells with a single central HADA focus representing a resolved septum). HADA labelling is increased in the centre of late-stage-dividing cells (Welch's t-test, P < 0.05). Localization of FacZ–mCherry does not change significantly as the septum resolves (Kolmogorov–Smirnov test, P > 0.05). d, Graphs of intensity profile scans measuring fluorescence intensity along peripheral arcs centred on the periseptum for early- and late-stage-dividing cells. e,f, Graphs of intensity profile scans of WT cells stained with HADA (light and dark blue) and Nile red (light and dark grey) imaged and analysed as in d and e, respectively. Perpendicular intensity profiles (c and e) were normalized from 0 to 1 for each cell. Peripheral intensity profiles (d and f) were interpolated using MATLAB, and intensity profiles were normalized from 0 to 1 for each fluorescence channel within each experiment, to facilitate comparison of cells of different sizes (c, n = 50 cells; d, n = 100 cells; e, n = 24 cells; f, n = 54 cells).
Source data
A role for FacZ in controlling Z-ring formation
To investigate what aspect of cell division FacZ participates in, we used Tn-seq to identify synthetic lethal partners. Transposon libraries were generated in wild-type and ΔfacZ cells, and the insertion profiles were compared (Supplementary Table 6). Insertion mutations were strongly depleted from many genes in the ∆facZ background relative to the wild type, with 102 loci bearing a 90% or greater reduction in insertions. Synthetic lethal interactions were identified for genes implicated in several biological pathways. However, many of the genetic interactions were with genes known to be required for cell wall biogenesis and/or cell division (Supplementary Table 7). The ezrA gene encoding a regulator of Z-ring formation27,28,29 was among these hits (67.6-fold depletion in ∆facZ, P < 0.005), suggesting that EzrA is essential in the absence of FacZ (Fig. 5a). We confirmed this synthetic lethal relationship by constructing an EzrA depletion strain and showing that EzrA depletion is lethal in a ΔfacZ background (Fig. 5b). Notably, mutants lacking either ezrA or facZ show increased mislocalization of FtsZ–GFP (Extended Data Fig. 3c,d), and both single mutants are hypersensitive to PC190723 (Fig. 5c), a chemical inhibitor of cell division that promotes hyper-stabilization of FtsZ filament bundles in Firmicutes30. Based on these genetic results, we infer that FacZ may play a role in controlling Z-ring formation as part of a parallel pathway that is partially redundant with EzrA.
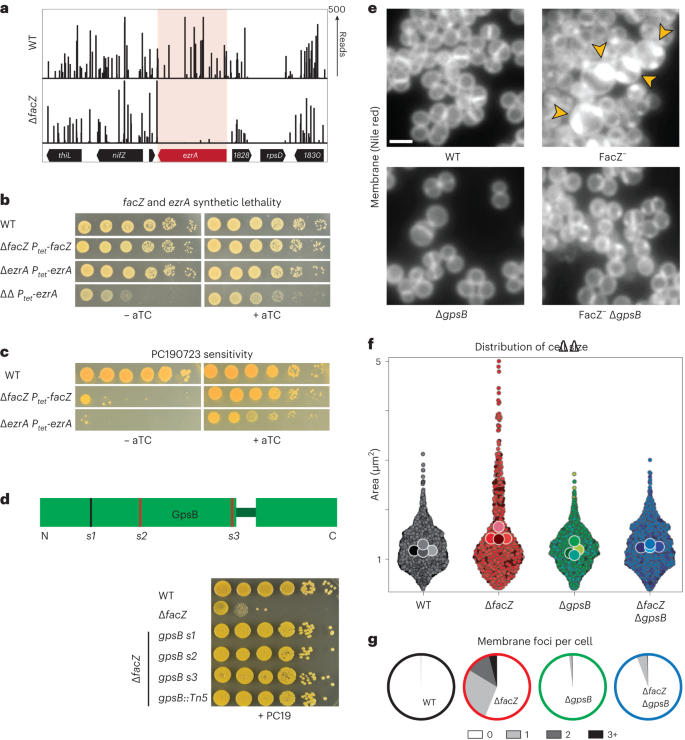
a, Transposon insertion profiles of the ezrA locus in strains aTB015 [WT] and aTB259 [∆facZ]. b, Spot titre of cultures of aTB003 [WT], aTB372 [∆facZ Ptet-facZ], aTP481 [∆ezrA Ptet-ezrA] and aTB378 [∆facZ ∆ezrA Ptet-facZ]. Cells were normalized to OD600 = 1.0, serially diluted and spotted on TSA agar with or without an aTC inducer (50 ng ml−1). c, Spot titre of aTB003 [WT], aTB372 [∆facZ Ptet-facZ] and aTP481 [∆ezrA Ptet-ezrA] as in b except that the plates contained PC190723 (100 ng ml−1). d, Top: Diagram showing the location of mutations in gpsB that suppress the PC190723 sensitivity of a ∆facZ mutant. Suppressor mutations are mapped onto a diagram of the two folded domains of GpsB (lines indicate position of mutations, with red lines indicating mutations generating premature stop codons). Bottom: Cultures of strains aTB003 [WT], aTB251 [∆facZ], aTB453 [∆facZ gpsB-s1], aTB476 [∆facZ gpsB-s2], aTB478 [∆facZ gpsB-s3] and aTB497 [∆facZ gpsB::Tn] were OD normalized and spotted on TSA supplemented with PC190723 (100 ng ml−1) as in b. e, Representative fluorescence images of aTB003 [WT], aTB372 [∆facZ Ptet-facZ], aTB525 [∆gpsB] and aTB540 [∆gpsB ∆facZ Ptet-facZ]. Strains were grown to mid-log phase without induction of facZ, and membranes were stained with Nile red (Extended Data Fig. 6). Yellow arrowheads highlight membrane defects. Scale bar = 2 µm. The scale bar applies to all images in e. f,g, Cultures of aTB521 [WT], aTB527 [∆facZ], aTB529 [∆gpsB] and aTB542 [∆facZ ∆gpsB] constitutively expressing cytoplasmic red fluorescent protein from pKK30 were labelled with TMA–DPH in mid-log phase and imaged on 2% agarose pads. f, Violin plots showing the cell area of indicated strains based on cytoplasmic fluorescence (WT, n = 1,760 cells; ∆facZ, n = 701 cells; ∆gpsB, n = 1,544 cells; ∆facZ ∆gpsB, n = 1,217 cells). Cell shape was quantified with MicrobeJ (Methods). g, The number of aberrant membrane foci per cell was quantified for display in pie charts (WT, n = 791 cells; ∆facZ, n = 400 cells; ∆gpsB, n = 998 cells; ∆facZ ∆gpsB, n = 721 cells).
Source data
FacZ is conserved in many Firmicutes, including Bacillus subtilis (Extended Data Fig. 5a–c). We previously found that inactivation of the B. subtilis homologue of FacZ (BsFacZ, formerly called YtxG) resulted in aberrant membrane invaginations and caused defects in sporulation31. Our results in S. aureus prompted us to reinvestigate the effect of inactivating BsFacZ in vegetative B. subtilis cells. We confirmed that cells deleted for BsfacZ show large, aberrant membrane invaginations via Nile red membrane labelling (Extended Data Fig. 5d). In addition, we found that BsFacZ inactivation caused misplaced FtsZ–GFP structures and led to an increase in cell length, consistent with a division defect (Extended Data Fig. 5e–h). Thus, FacZ may have a conserved role in promoting proper Z-ring and septum formation among members of the Firmicutes.
FacZ functions as a GpsB antagonist
To further explore the role of FacZ in division, we selected for suppressor mutations that overcome the hypersensitivity of S. aureus ∆facZ cells to PC190723. Whole-genome sequencing of the isolated suppressors identified three unique mutations in gpsB, a gene encoding a conserved Firmicute-associated protein with a role in envelope biogenesis32. Notably, GpsB in S. aureus has been shown to interact directly with FtsZ via a GpsB-interacting motif in FtsZ33,34 that is not shared by FtsZ from B. subtilis35. Thus, GpsB is thought to play a direct role in cell division in staphylococci that may be unique to this group of organisms. The gpsB lesions in the ∆facZ suppressors included an in-frame duplication of residues 21–24 and two deletions causing premature stop codons at residues 34 and 66, all of which are likely to disrupt GpsB function (Fig. 5d). Although GpsB has been previously described as essential in S. aureus33, Tn-seq analyses from our labs and others have identified Tn mutants throughout gpsB in several strains of S. aureus12,36, and the Nebraska Transposon Mutant Library (NTML) collection of non-essential S. aureus genes contains a Tn mutant in gpsB37. Accordingly, we constructed a kanamycin-marked ∆gpsB (∆gpsB::kanR) strain. Both this insertion–deletion and the Tn-inactivated allele from the NTML collection37 were readily transduced between strains, indicating that gpsB is dispensable for growth in our conditions. As expected from the suppressor analysis, inactivation of gpsB alleviated the PC190723 hypersensitivity of ∆facZ cells (Fig. 5d). Importantly, GpsB inactivation also largely suppressed the cell size and membrane invagination defects caused by deletion of facZ (Fig. 5e–g and Extended Data Fig. 6a–d), and partially rescued the synthetic lethal phenotype of ∆facZ ∆ezrA cells (Extended Data Fig. 6h). These results suggest that FacZ is required to antagonize GpsB function. In support of this idea, overexpression of gpsB enhanced the growth defect of the ∆facZ mutant (Extended Data Fig. 6i).
Localization of FacZ–mCherry and mNeon–GpsB fusions indicate that both proteins are present at the periseptal region in cells at early stages of division (Fig. 6a,b and Extended Data Fig. 6e–g). However, FacZ–mCherry remains at the periseptum in later stages of division while mNeon–GpsB localizes throughout the septum (Fig. 6a,b and Extended Data Fig. 6e–g). Notably, the localization of mNeon–GpsB was altered upon inactivation of FacZ, with the fusion enriching at many sites throughout the cell coincident with areas of aberrant membrane invagination (Fig. 6c). These findings suggest that FacZ functions by constraining GpsB localization and activity to a single site to promote normal division.
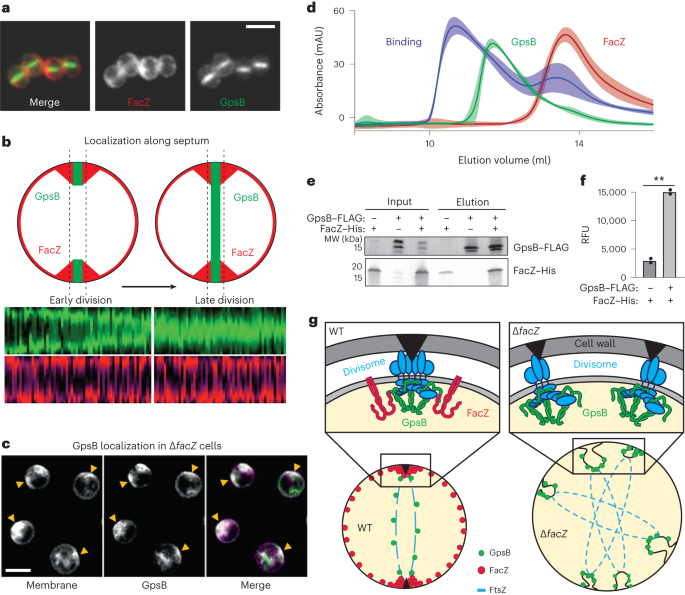
a, Representative images of aTB517 [∆facZ ∆gpsB Ptet-gpsB-mNeon Pspac-facZ-mCherry] grown in the presence of aTC (50 ng ml−1) and IPTG (25 ng ml−1) and imaged by fluorescence microscopy (scale bar = 2 µm; the scale bar applies to all images in a). b, Graphs showing the fluorescence intensity of FacZ–mCherry (light and dark red) and GpsB–mNeon (light and dark green) along lines parallel with the septum. Cells were separated into two groups based on the GpsB–mNeon signal: cells with discontinuous GpsB foci were considered early-division cells (top left) whereas cells with a continuous GpsB–mNeon band at the septum were considered late-division cells (top right) (n ≥ 50 cells for each group). c, Representative deconvolved fluorescence images of GpsB–mNeon localization in ∆facZ cells (aTB519) labelled with Nile red (scale bar = 2 µm; the scale bar applies to all images in c). d, Gel filtration profiles of GpsB (1–75) alone (green), SUMO-3x-FacZ (127–146) alone (red) or a mixture of the two proteins (binding, blue). Elution profiles represent the average and standard deviation of six runs. e, Lysates were generated from strains expressing tagged variants of FacZ and GpsB [aTB411, 513 and 514]. Membrane fractions were solubilized, loaded onto α-FLAG (DYKDDDDK octamer) magnetic beads, washed and eluted. Input (membrane homogenate) and output (elution) were resolved by sodium dodecyl sulfate–polyacrylamide gel electrophoresis (SDS-PAGE) and blotted using α-His and α-FLAG antibodies (left). f, Fluorescence intensities of the FacZ bands from the blot in e and a replicate experiment (n = 2 biologically independent samples) were measured and relative fluorescence units (RFUs) were plotted (right) (**P = 0.0018). g, Schematic model of FacZ function within the divisome. Cell division is properly localized when FacZ is functional (left). In the absence of FacZ, GpsB is unregulated such that cell constriction is initiated at many sites (right). See text for details.
Source data
To investigate whether FacZ interacts with GpsB to control its function, we tested whether the two proteins associate in vivo. In S. aureus cells, 6xHis-tagged FacZ (FacZ–His) was co-expressed with FLAG-tagged GpsB (GpsB–FLAG). Immunoprecipitation of GpsB–FLAG with anti-FLAG resin resulted in the significant enrichment of FacZ–His in the eluted fraction (Fig. 6e,f and Extended Data Fig. 7f), indicating that the two proteins reside in a complex. To determine whether the interaction is direct, we further characterized their binding in a purified system. Previous crystal structures show that the N-terminal domain of GpsB forms a dimer that binds to small peptides of S. aureus FtsZ and PBP4 bearing a (S/T/N)RxxR(R/K) motif32,35. We reasoned that FacZ might interact with GpsB by a similar mechanism and found that it contains a canonical staphylococcal GpsB-binding motif at residues 134–139 (NRHYRR) (Extended Data Fig. 5c). Full-length FacZ was insoluble following overexpression. We therefore purified a small ubiquitin-like modifier-tagged (SUMO-tag) fusion bearing a 3× concatenation of residues 127–146 of FacZ (FacZ127–146) harbouring the putative GpsB-binding motif. Incubation of this fusion with the N-terminal domain of GpsB (GpsB1–75) resulted in a shift in the elution profile of the two proteins by size-exclusion chromatography, indicating that the two proteins interact directly (Fig. 6d). We also found that the arginine residues within the NRHYRR motif are collectively critical for FacZ–GpsB interaction, as the charge-swapped allele NDHYDD is unable to bind GpsB in vitro (Extended Data Fig. 7a,b). Importantly, a FacZ variant harbouring these same changes accumulated to wild-type levels in cells but did not complement the PC190723 sensitivity of a ∆...
Comments
Post a Comment